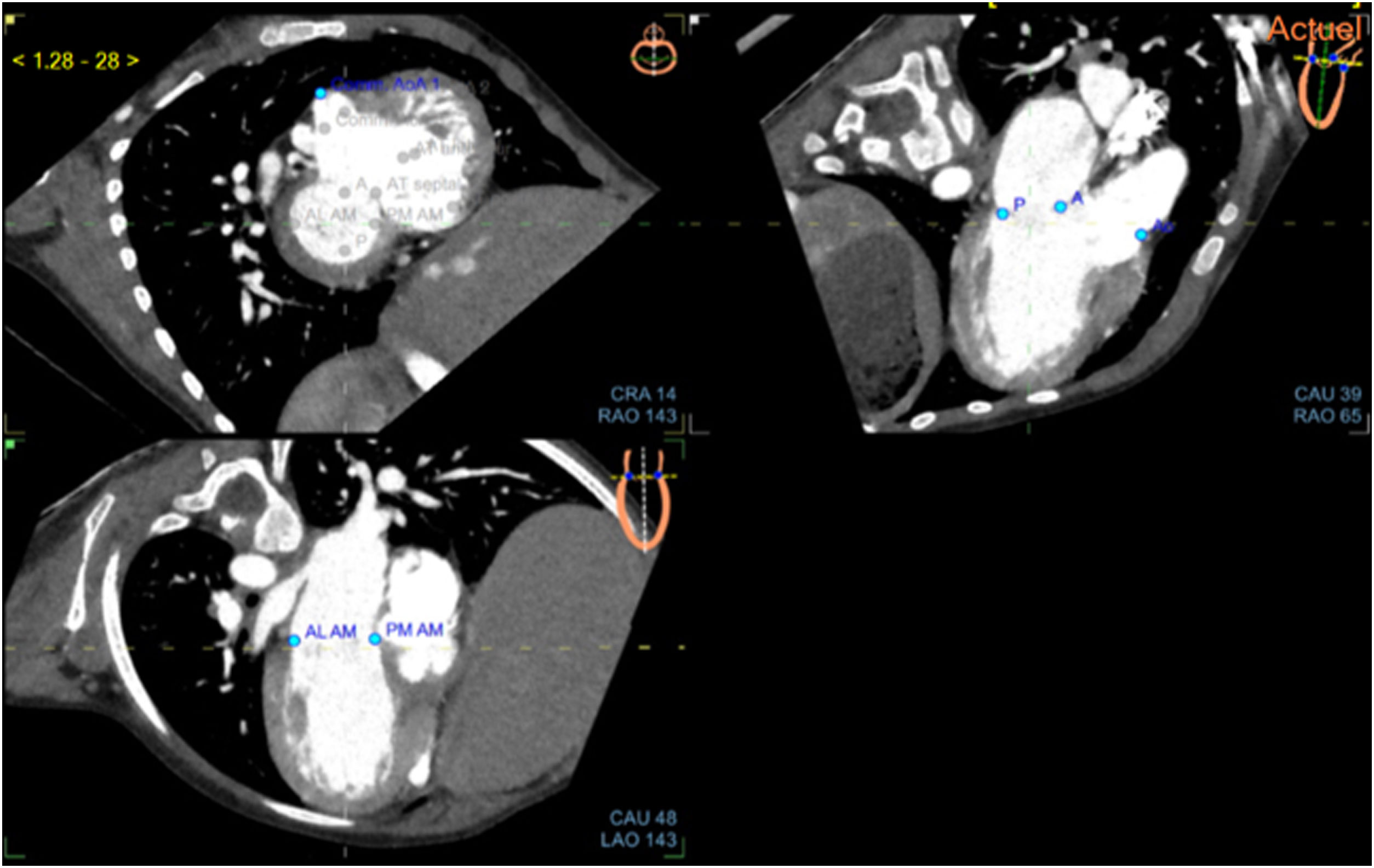
Diagnosis, management, and surgical decision-making in children and adults with congenital heart disease are largely based on echocardiography findings. A recent development in cardiac imaging is fusion of different imaging modalities. Our objective was to evaluate the feasibility of computed tomography (CT) and 3-dimensional (3D) transthoracic echocardiography (TTE) fusion in children and adults with congenital heart disease.
MethodsWe prospectively included 14 patients, 13 of whom had congenital heart disease, and who underwent both CT and 3D TTE as part of their usual follow-up. We described the steps required to complete the fusion process (alignment, landmarks, and superimposition), navigation, and image evaluation.
ResultsMedian age was 9.5 [2.7-15.7] years, 57% were male, and median body surface area was 0.9 m2 [0.6-1.7]. Congenital heart disease was classified as simple (n=4, 29%), moderate (n=4, 29%), or complex (n=6, 42%). 3D TTE-CT fusion was successful in all patients. Median total time to complete the fusion process was 735 [628-1163] seconds, with no significant difference according to the degree of complexity of the defects. Landmarks were significantly modified in complex congenital heart disease.
ConclusionsWe established the feasibility and accuracy of 3D TTE-CT fusion in a population of children and adults with a variety of congenital heart diseases. The simultaneous visualization of many intracardiac structures may help to understand the anatomical features of congenital heart disease without limitations regarding age, weight, or complexity of the congenital defects.
Keywords
Echocardiography is the cornerstone of the diagnosis, management, and surgical decision-making in children and adults with congenital heart disease (CHD). Transthoracic echocardiography (TTE) provides information on the cardiac valves, notably regarding stenosis or regurgitation, intracardiac shunts, and on ventricular function and volumes. Three-dimensional (3D) TTE has recently emerged as a higher-resolution tool capable of evaluating intracardiac anatomy with greater accuracy.1 Intracardiac projections with different views such as en-face views provide diagnostic information that is difficult to obtain with 2D TTE or cardiac computed tomography (CT).2 However, 3D-TTE is difficult to use in practice and requires specific training. CT is a useful complementary investigation, notably for assessing congenital abnormalities of the blood vessels. When CT is used by experienced teams, high resolution can be obtained and radiation exposure minimized.3
One of the most recent developments in cardiac imaging is the fusion of different modalities. In children and adults with CHD, fusion between transesophageal echocardiography and fluoroscopy during catheterization provides a better understanding of the complex cardiac anatomy. This method helps to guide interventional procedures, such as the percutaneous closure of atrial septal defects or ventricular septal defects.4,5 Fusion between a preprocedural CT and fluoroscopy is also useful to guide percutaneous interventions on abnormal vessels, such as coarctation stenting or aortic endoprosthesis implantation to exclude an aortic aneurysm.6,7
Echocardiography-CT fusion is currently under evaluation. Transesophageal echocardiography-CT fusion has recently been used to guide transcatheter tricuspid valve annuloplasty8 or to close left appendages.9 TTE-CT fusion has also been evaluated in adults with coronary artery disease.10,11 To date, no information has been published on the feasibility of TTE-CT fusion in children or adults with CHD. A tool that would facilitate the combined evaluation of the intracardiac and extracardiac anatomical features would be valuable to plan cardiac surgery in individual patients with CHD. However, the wide spectrum of CHDs represents a technical challenge for TTE-CT fusion.
The main objective of this preliminary study was to assess the feasibility of TTE-CT fusion in a population of adults and children with a wide variety of CHD.
METHODSIn a single tertiary center, we consecutively included 14 patients who underwent both a CT and a TTE as part of their usual care, over a 6-week period from July to August 2020. We used the score designed by the American Heart Association and American College of Cardiology12 to classify CHDs as simple, moderate, or complex. Mean heart rate during echocardiography was noted. Patients were informed about the study design and consent was obtained. This study was registered in our institution (FUSIONSCANECHOCC n°2022-565) and approved by the institutional review board.
Computed tomography imagingImages were acquired with a 512-slice CT (Revolution CT, General Electric Healthcare, USA). The usual premedication protocol was followed, and no beta-blockers were used. Irradiation parameters were previously optimized by the radioprotection unit to achieve a good image quality/irradiation ratio (0.60mm collimation, 0.625mm reconstruction interval, and 70-80kV). A prospective electrocardiogram-triggered axial technique was used within a single heartbeat for all patients, with a systolic acquisition from 40% to 50% of the RR interval. Contrast agent (iodine concentration, 300mg) was injected into a peripheral vein in a dosage of 2mL/kg for patients up to 40kg in body weight and 1.5mL/kg for patients weighing 10 to 40kg, at a flow rate of 0.6 to 4mL/s according to body weight. An iterative reconstruction algorithm (Asir-V [General Electric Healthcare] with 50% strength) and specific reconstruction software reducing coronary motion artifacts (SnapShot Freeze, General Electric Healthcare) were used. The dose delivered to patients was evaluated in terms of the CT dose index and dose-length product (DLP). Effective doses (EDs) were derived from chest conversion factors using the following formula: ED (mSv)=DLP (mGy x cm) x k (mSv x mGy-1 x cm-1).
Transthoracic echocardiographyStudies were acquired using a Vivid E95 GE (General Electric Healthcare, Vingmed Ultrasound, Norway) with an adult probe fitted with a matrix array transducer (S4). At the end of the exam, a full-volume 3D heart imaging was obtained over 3 cardiac cycles using ECG-gated acquisition of a large 3D pyramidal volume. This step consisted of positioning the S4 probe for a 4-chamber apical view acquisition and selecting the 3D program. The entire left ventricular cavity was included within the pyramidal scan volume and imaging depth was minimized to maximize the frame rate and the axial and temporal resolution. Gain settings were optimized for endocardial visualization. No medication was given to control the heart rate.
Image fusionTTE-CT fusion was achieved using the first release of the commercially available General Electrics Fusion Software. The steps required to complete the process are listed below.
Image importationCT slices were selected and extracted in Digital and Imaging Communications in Medicine format from our Picture Archiving and Communication System. They were manually imported, with a USB key or a CD, into a dedicated ultrasound machine (Vivid E95, General Electrics) for postprocessing and fusion. CT datasets from other manufacturers could also be imported. TTE images were acquired on the dedicated ultrasound machine or manually imported in raw format. Then, the CT and TTE datasets were merged manually within the same patient ID prior to postprocessing. When the 2 datasets were combined, the fusion process could begin. The time taken to complete these preliminary steps was not recorded.
Fusion processThe fusion process started with CT alignments. No anatomical knowledge was required to realize the alignments and the placement of landmarks. The objective was to reproduce a reference image visible at the top right of the screen. Three standardized views had to be obtained by moving 3 orthogonal alignments (yellow, green, and white lines) for each valve. For the mitral valve, the 3 reference views were the short axis view (figure 1A); apical 3-chamber view with the left atrium, left ventricle, and aorta (figure 1B); and apical 2-chamber view with the left atrium and left ventricle (figure 1C). For the aortic valve, the 3 reference views were the horizontal (figure 2A), sagittal (figure 2B), and coronal (figure 2C) views. Finally, for the tricuspid valve, the 3 reference views were the short axis view, apical 2-chamber view, and apical 4-chamber view with the right atrium and right ventricle. After validation of this step, 9 CT anatomical landmarks were placed according to the reference images proposed by the software (3 for each valve, figure 1A,B and figure 2A). The anatomical landmarks were placed on the anterior, posterior, anterolateral, and posteromedial parts of the mitral valve and on the aorta for the mitral valve; on the 3 commissures for the aortic valve; and on the anterior, posterior, lateral, and septal parts of the tricuspid annulus for the tricuspid valve.
Step 1: Alignments and landmarks of the mitral valve on CT. A: short axis view. B: apical 3-chamber view. C: apical 2-chamber view. Note the orthogonal alignments in each panel (white, yellow, and green lines) focused on the mitral valve. The blue spots are the landmarks (A, anterior; P, posterior; Ao, aorta; AL, anterolateral; PM, posteromedial) to be placed according to the reference image at the top right of each panel. CT, computed tomography.
Step 2: Alignments and landmarks of the aortic valve on CT. A: horizontal view. B: sagittal view. C: coronal view. Note the orthogonal alignments in each panel (white, yellow, and green lines) focused on the aortic valve. In panel A, the blue spots are the landmarks to be placed according to the reference image at the top right and correspond to the commissures. The first landmark is the left-right commissure (1), and the other 2 commissures are then seen in the counterclockwise direction. The previous landmarks are present but grayed. Ao, aorta; CT, computed tomography.
The same procedure was performed with the TTE images. The software is formatted for transesophageal echocardiography and not for TTE. The echocardiography views had to be reversed before the performance of alignment. A valve had to be chosen for alignment (mitral, tricuspid or aortic valve). The mitral valve was chosen for all patients in our study. Three orthogonal mitral valve alignments were placed (figure 3) and 3 mitral valve landmarks were then identified (figure 4B,C).
Step 3: Alignments of the mitral valve on TTE. A: 3D TTE corresponding to panel D. B: apical 3-chamber view. C: apical 2-chamber view. D: short axis view. Note the orthogonal alignments in each panel (white, yellow, and green lines) focused on the mitral valve according to the reference image at the top right of each panel. TTE, transthoracic echocardiography.
Step 4: Landmarks of the mitral valve on TTE. A: 3D TTE corresponding to panel D. B: apical 3-chamber view. C: apical 2-chamber view. D: short axis view. The blue spots are the landmarks (A, anterior; P, posterior; Ao, aorta; AL, anterolateral; PM, posteromedial) to be placed on panels B and C according to the reference image at the top right of each panel. TTE, transthoracic echocardiography.
CT and TTE alignments were described as easy or difficult for each patient. This easy vs difficult description was subjective but relied on a single operator who performed all these exams without prior specific training. An easy alignment was perceived as short, without spatial orientation difficulty even when the echocardiography views had to be reversed. It was also noted when the landmarks had to be placed differently from the reference landmarks due to the specific anatomy of some complex CHD (blue spots on the reduced image at the top right of the screen) eg, atrioventricular or ventriculoarterial discordance, different orientation of the valves. To check that fusion was achieved, the operator performed a visual subjective assessment of the quality of matching of the TTE and CT layers (figure 5). The quality of the superimposition was scored from 1 to 10. Dynamic transparency of the echocardiographic view over the CT scan view was used for this step (video 1 of the supplementary data). If necessary, the TTE imaging axis could be moved manually on the CT image to adjust the accuracy of the superimposition. For each step, the time required, and difficulty scored on 10 were recorded. The accuracy of the process was defined by the quality of superimposition.
Step 6: Validation of fusion imaging. A: short axis view. B: apical 3-chamber view. C: apical 2-chamber view. Simultaneous visualization of the CT images with the overlay of the TTE images. The TTE layer can be moved if necessary, so that it matches the CT images perfectly. CT, computed tomography; TTE, transthoracic echocardiography.
After superimposition, navigation in the fused image could start. Navigation allowed the evaluation of a structure using many modalities at the same time: a) 2D by 2CT: 2 video clips with 2D TTE and 2 images with CT in the same orientation (video 2 of the supplementary data); b) 3D by 3CT: 1 3D TTE view and 3 different CT views. The 3D TTE view (top left corner) is presented in the same orientation as one of the CT views (bottom right), and the reference CT view can be changed if desired (video 3 and 4 of the supplementary data); c) CT, 3D TTE, and 2D TTE views at the same time.
Statistical analysisContinuous variables are described as median [interquartile range]. Comparisons between measurements in patients in the 3 CHD complexity categories were performed using the nonparametric Mann-Whitney test. For all analyses, P values> .05 were considered significant. The statistical analyses were performed using Stata software, version 11.2 (StataCorp, USA).
RESULTSWe consecutively included 13 patients with CHD and 1 patient with a structurally normal heart, Wolff-Parkinson-White syndrome, and suspicion of coronary artery anomaly ruled out by CT. Their main features are described in table 1. No patients were excluded prior to fusion imaging.
Patients’ demographic characteristics
Variable | ValuesN=14 |
---|---|
Age, y | 9.5 [2.7-15.7] |
Male sex | 8 (57) |
Weight, kg | 22.8 [12.6-59.0] |
Height, cm | 127 [89-165] |
BSA, m2 | 0.9 [0.6-1.7] |
Heart rate, bpm | 87 [78-120] |
Type of CHD | |
Simple | 4 (29) |
Moderate | 4 (29) |
Complex | 6 (42) |
Fusion indication | |
Catheterization | 4 (29) |
Surgery | 8 (57) |
Diagnosis | 2 (14) |
Fusion feasibility | 14 (100) |
Unless otherwise indicated, data are expressed as No. (%) or median [interquartile range]. BSA, body surface area; CHD, congenital heart disease.
Fusion between 3D TTE and CT was feasible in all patients. We started with mitral valve alignment of the CT images. This step was easy in 12 (85.7%) patients in the short axis view, 9 (64.3%) patients in the apical 3-chamber view, and in all patients in the apical 2-chamber view. Alignment in the short axis view was difficult in patient no. 2 with abnormal pulmonary venous return because of the absence of the interatrial septum and in patient no. 9 due to considerable left atrium dilation that altered the landmarks between the left atrium and the left ventricle. Alignment in the apical 3-chamber view was difficult in 5 patients due to anterior malposition of the aorta. Once mitral valve alignment was achieved, alignment of the tricuspid valve and aortic valve was easy.
In 8 (57.1%) patients, the CT landmarks were placed differently from the reference landmarks for the mitral valve and tricuspid valve because of atrioventricular discordance, and for the aortic valve because of malposition of the aorta (double outlet right ventricle, dextro- or levotransposition of the great arteries). However, the process was feasible when the tricuspid valve was considered as the mitral valve in the case of atrioventricular discordance and the pulmonary valve as the aortic valve in case of transposition of great arteries. The tricuspid valve landmark was difficult to locate in 2 patients, 1 with right ventricle dilation and the other with corrected-transposition of great arteries and Ebstein anomaly.
For TTE alignments, mitral valve alignment in the short axis view was difficult in 3 (21.4%) patients because of a ventricular septal defect or left atrium enlargement. Mitral valve alignment in the apical 3-chamber view was difficult in 7 (50%) patients due to malposition of the great vessels and reduced echogenicity of the anterior structures. In 7 (50%) patients, the TTE landmarks were placed differently from the reference landmarks due to valve discordance.
Accuracy of the processTable 2 describes the fusion process according to CHD complexity. Superimposition quality was good with a median score of 8.5/10 (figure 5). A slight modification of the TTE layer on the CT image was required in 6 (43%) patients, of whom 2 had moderate CHD and 4 complex CHD.
Computed tomography and 3D TTE fusion process in simple, moderate, and complex congenital heart diseases
Variable | Simple/moderate n | Complex n | P |
---|---|---|---|
Modification of the superimposition | 2 | 4 | .277 |
CT alignment | ns | ||
Modified CT landmark | 2 | 6 | .010 |
VM | 1 | 1 | .692 |
VT | 0 | 2 | .165 |
VA | 1 | 5 | .026 |
CT difficulty, scored 1 to 10 | 2.0 [1.0-3.5] | 5.5 [3.0-7.0] | .051 |
CT time, s | 376 [330-467] | 540 [319-820] | .302 |
TTE alignment | ns | ||
Modified TTE landmark | 1 | 6 | .005 |
VM | 1 | 1 | 1 |
VT | 0 | 2 | .165 |
VA | 1 | 5 | .026 |
TTE difficulty, scored 1 to 10 | 2.0 [1.0-3.0] | 6.5 [5.0-7.0] | .005 |
TTE time, s | 293 [218-341] | 657 [275-735] | .093 |
Total fusion time, s | 696 [632-746] | 1320 [594-1555] | .272 |
The data are expressed as No. or median [interquartile range]. CT, computed tomography; TTE, transthoracic echocardiography; VA, aortic valve; VM, mitral valve; VT, tricuspid valve.
Median times were 423 [329-558] seconds for the CT process and 302 [271-597] seconds for the TTE process. Median total time required to complete the fusion process was 735 [628-1163] seconds. Total time to obtain fusion images was longer in patients with complex CHD, although this difference was not significant (P=0.272). Landmarks were significantly modified in patients with complex CHD.
Description of cases and utility of fusion.Table 3 describes the main features in the 14 patients. In the patient with a normal heart, fusion was simple and navigation in all planes and modalities was informative (video 3 of the supplementary data). In selected patients with complex CHDs, navigation in the heart using multiple modalities simultaneously appeared as useful for the diagnosis or surgical planning, combining different views at the same time. As an example, in a patient with double outlet right ventricle, a single panel of a fusion image visualized the ventricular septal defect, the conal septum, the ventriculoarterial relationships, the size of the right ventricle, and the absence of an overriding or straddling atrioventricular valve (figure 6, and videos 2 and 4 of the supplementary data). On video 2, we note the position of the pulmonary artery suggesting that a direct connection from the right ventricle to it is not feasible. 2D TTE shows no obstruction in the ventricular septal defect for a left ventricle-aorta connection. Video 4 of the supplementary data showed that there was no straddling of the atrioventricular valves across the large doubly committed ventricular septal defect. CT was useful in the short axis view to visualize the pulmonary artery, which wasn’t seen on the 3D TTE.
Description of the 14 study patients
Age, y | Weight, kg | Heart rate, bpm | Type of CHD | |
---|---|---|---|---|
Patient 1 | 11 | 46 | 83 | Wolff-Parkinson-White syndrome |
Patient 2 | 0.9 | 6.8 | 151 | DORV, D-TGA, anomalous systemic return, PS |
Patient 3 | 3 | 14 | 90 | DORV, PS |
Patient 4 | 21 | 67 | 72 | TOF |
Patient 5 | 1 | 7.5 | 129 | Multiple VSD |
Patient 6 | 7 | 22.8 | 80 | AVSD, PS |
Patient 7 | 11 | 40 | 82 | ASD |
Patient 8 | 20 | 65.1 | 78 | Right pulmonary vein stenosis |
Patient 9 | 11 | 22.8 | 120 | RCM |
Patient 10 | 15 | 79.9 | 64 | Congenital anomaly of aortic valve |
Patient 11 | 6 | 19.5 | 118 | Corrected TGA, VSD, PS |
Patient 12 | 18 | 59 | 70 | D-TGA, VSD, PS, Total cavo-pulmonary derivation |
Patient 13 | 1 | 6.8 | 124 | L-TGA, multiple VSD, PS, ventricle superposition |
Patient 14 | 2 | 12.6 | 101 | DORV, L-TGA |
AVSD, atrio-ventricular septal defect; ASD, atrial septal defect; DORV, double outlet right ventricle; PS, pulmonary stenosis; RCM, restrictive cardiomyopathy; TOF, tetralogy of Fallot; TGA, transposition of great arteries; VSD, ventricular septal defect.
(central illustration). Navigation in fusion imaging in a patient with a double outlet right ventricle. A: CT mitral valve alignment on the short axis, apical 3-chamber, and apical 2-chamber views. B: CT aortic valve alignment and landmarks on the horizontal, coronal, and sagittal views. C: CT tricuspid valve alignment and landmarks on the short axis, apical 2-chamber, and 4-chamber views. D: 3D TTE showing the double outlet right ventricle, alignment, and mitral valve landmarks. The patient had a double outlet right ventricle. Note the ventriculoarterial disposition with an anterior aorta and the doubly committed ventricular septal defect, absence of a conal septum between the great vessels, hypoplasia of the pulmonary annulus, and absence of straddling or overriding of the atrioventricular valves. CT, computed tomography; TTE, transthoracic echocardiography.
This study demonstrates the feasibility of TTE-CT fusion in 13 patients with CHD of various complexity. TTE-CT fusion combines the simultaneous analysis of 2D and 3D TTE images with CT in synchronized views. The application of fusion imaging technology to CHD is still limited. To the best of our knowledge, this is the first study showing the feasibility of TTE-CT fusion imaging in children and adults with CHD. We focused on the feasibility of CT fusion with TTE images since preprocedural transesophageal echocardiography is rarely performed in children. We demonstrate that TTE-CT fusion was feasible in patients covering broad age and weight ranges and having different CHD complexities. We analyzed many anatomical variations in CHD, namely: atrioventricular discordance, ventriculoarterial discordance, malposition of the great arteries, ventriclular superposition, mitral and tricuspid valve anomalies, and aortic and pulmonary valve anomalies. The fusion process was longer in patients with complex CHD than in those with simple or moderate CHD. This is partly explained by the need to modify the landmarks according to the patient's anatomy. The difficulty was greater for TTE as alignment was more challenging to obtain because of the lack of echogenicity of very anterior cardiac structures.
In simple and moderate CHD, fusion imaging was informative, for example in a patient with multiple ventricular septal defects: a persistent residual ventricular septal defect after surgery was localized by fusion imaging and was then closed percutaneously. Fusion imaging seems highly informative for complex CHD, particularly when the ventriculoarterial connections are abnormal. An accurate description of the relationships between intracardiac structures has a direct impact on the surgical approach. We were, for example, able to display simultaneously the CT and 3D TTE views of a double outlet right ventricle, showing to surgeons the spatial relationships between atrioventricular valves, the ventricular septal defect, and the great vessels.
Fusion imaging is now mostly used to guide interventional procedures in acquired and CHD. First, fusion between fluoroscopic images and transesophageal echocardiography has been used to guide left atrial appendage closure, percutaneous mitral valvuloplasty, percutaneous sinus venosus or ostium secundum atrial septal defect closure, percutaneous ventricular septal defect closure or perivalvular leak closure.5,8,13-16 Second, fluoroscopy-CT fusion was useful to guide coarctation or pulmonary artery stenting and percutaneous valve implantations.15 Third, transesophageal echocardiography-CT fusion improved the assessment of left atrial appendage anatomy, helping catheter orientation, thereby reducing procedure time9 and facilitated transcatheter tricuspid valve annuloplasty, assessing the severity and mechanism of tricuspid valve regurgitation, as well as of the size of the tricuspid annulus and its spatial relationship with the right coronary artery.10
Although the applications of fusion imaging for interventional procedures seem to be undergoing rapid development, few studies have focused on improving diagnosis and cardiac surgery guidance in patients with CHD, which remain challenging. A better understanding of the complex spatial relationships and anatomy in patients with CHD may avoid unexpected findings and decrease operating time. There is clear evidence that printing of 3D models can affect surgical decision-making, particularly regarding biventricular repair in complex CHD.16 However, dynamic structures such as cardiac valves and flows cannot be analyzed in these models.
When discussing patient cases before an intervention, TTE (2D, 3D) and CT images are presented separately. The anatomical analysis is based on the sonographer who performed the ultrasound. Some cases are challenging and therefore there is increasing interest in image fusion to combine the strengths of these modalities during staff meetings with cardiac surgeons, showing the regions of interest and the navigation into the heart with a simultaneous hemodynamic and anatomic evaluation. Despite the anatomical variations in CHD, TTE-CT fusion does not require any special skills in 3D TTE but allows the display of 3D images of optimal quality. Only a full-volume 3D heart acquisition is necessary and, consequently, the learning curve is limited. Moreover, the software allows visualization of CT and 2D echo images on the same axis. These images are produced by multiplanar navigation on the volume dataset, do not depend on the incidence of acquisition of the sonographer and allow visualization of a dynamic 2D echo plane as requested by surgeons or interventionalist during a meeting.
LimitationsAfter importation of CT and acquisition of TTE images, the median total time required to complete the fusion process was more than 12minutes. This delay did not include the time required to import CT scan data. Importing the CT data from the local network will decrease overall processing time. Moreover, there was a clear trend toward a longer time to perform the fusion in patients with complex CHD due to the modification of the landmarks. This means that the process is manually corrected and expertise in CHD is required to adjust landmarks. The process of fusion, the alignments, and the landmarks were not saved in the ultrasound machine. When the process is ended, previous fusion images cannot be recovered, which clearly increases the work time. Furthermore, this tool is formatted for transesophageal echocardiography. Improvements in the TTE-CT fusion process would simplify its use in everyday clinical practice. Adding 3D color flow Doppler would be useful. If fusion can be achieved with any CT images, TTE images need to be performed on a GE ultrasound machine.
The feasibility of TTE-CT fusion in smaller children was not assessed because of the unavailability of a dedicated pediatric probe at the time of this study. A limited variety of CHD is presented because we included only patients who underwent both CT and TTE as part of their usual care. Despite this, we presented many anatomical variations of CHD. Only 3 adults were included, and the feasibility of 3D TTE-CT fusion imaging should be more precisely assessed in adults with CHD who may have a limited acoustic window. Nowadays, the dedicated pediatric matrix array transducer allows the feasibility of 3D TTE-CT fusion even in smaller children.
The software allows mainly simultaneous visualization of CT and 2D or 3D images in the same incidence. Fusion of CT and echo images are only produced at the end of the alignments step to check the accuracy of merging. Finally, the aim of the study was to depict the feasibility of TTE-CT fusion imaging in CHD patients, including those with complex CHD. Its usefulness and added value in clinical practice remain to be demonstrated, particularly in complex CHD and should be compared with other innovative imaging modalities such as 4D flow magnetic resonance imaging and 3D printing.
CONCLUSIONSThe feasibility of 3D TTE-CT fusion imaging is demonstrated in a population of patients covering a broad age range and having a wide variety of CHD. TTE-CT fusion allows the simultaneous visualization of many intracardiac structures and may aid understanding of the complex anatomy of CHD.
In this preliminary study, we focused on and showed the feasibility of the TTE-CT fusion in a small sample of CHD patients with various anatomy. Whether this tool provides useful additional information, improves diagnosis, and reduces surgical complications compared with standardized protocols needs to be studied in larger cohorts. In addition, the usefulness of TTE-CT fusion for communication and educational purposes should be evaluated.
- -
Fusion of different modalities is one of the most recent developments in cardiac imaging.
- -
A tool that would facilitate the combined evaluation of the intracardiac and extracardiac anatomical features would be valuable to plan cardiac surgery in individual patients with CHD.
- -
Little is known about the feasibility of fusion between transthoracic echocardiography and cardiac tomography in children or adults with CHDs.
- -
Fusion between computed tomography and 3D transthoracic echocardiography is feasible in children and adults with CHD whatever the degree of complexity of the cardiopathy.
- -
A modified protocol is required to merge the dataset of patients with complex CHDs including ventricular-arterial connection anomalies.
- -
Whether this tool provides useful additional information and improves the pretherapeutic assessment compared with standardized protocols should be studied in larger cohorts.
No funding.
AUTHORS’ CONTRIBUTIONSSubstantial contributions to the conception and design: E. Fournier, C. Batteux, M. Mostefa-Kara, S. Hascoët. Substantial contributions to the acquisition of data, analysis, and interpretation of data: E. Fournier, C. Batteux, M. Mostefa-Kara, A. Azarine, A. Sigal-Cinqualbre. Manuscript drafting: E. Fournier, E. Valdeolmillos, S. Hascoët. Critical revision for important intellectual content: E. Fournier, C. Batteux, M. Mostefa-Kara, E. Valdeolmillos, A. Maltret, S. Cohen, I. Van Aerschot, L. Guirgis, A. Azarine, A. Sigal-Cinqualbre, B. Provost, J. Radojevic-Liegeois, R. Rourssin, J. Zoghbi, E. Belli, S. Hascoët.
CONFLICTS OF INTERESTThe authors have no conflict of interest in relation with this study.
Supplementary data associated with this article can be found in the online version, at https://doi.org/10.1016/j.rec.2022.04.002