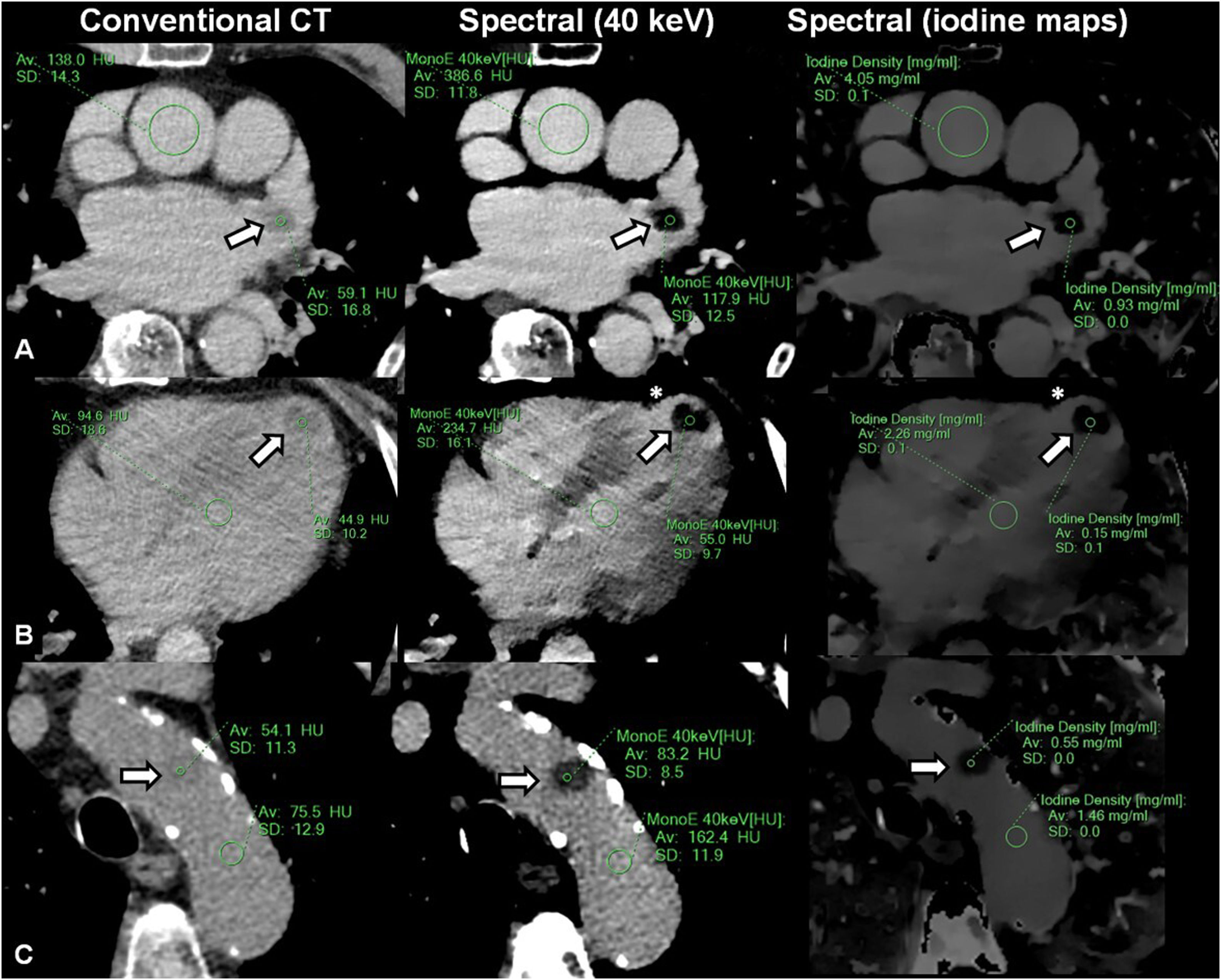
In the past decade, spectral computed tomography (CT) has emerged as a useful means to improve tissue characterization. However, few studies have explored its potential usefulness for the detection of cardiac and aortic thrombi.1–3 Furthermore, although delayed-phase imaging typically provides the most valuable datasets aimed at ruling out thrombi, there are scarce reports regarding threshold values for thrombotic sources.4 With the advent of dual-layer scanners that enable spectral routine acquisitions without protocol modifications, and in the context of acute ischemic stroke imaging, there is an expected increase in the number of scans with incidental thrombi. These scanners enable the availability of simultaneous multiparametric data including conventional CT images, monoenergetic imaging ranging from low (40keV) to high (200 keV) energy levels, and iodine density maps among others. Accordingly, we sought to evaluate the incremental value of monoenergetic and iodine density maps over conventional CT for the discrimination of thrombi in patients with acute ischemic stroke (AIS).
From July 2020, patients with suspected AIS admitted in our emergency department underwent a low-dose chest CT after CT angiography with the main goal of attempting to simultaneously rule out cardiovascular thrombotic complications using a dual-layer spectral CT (IQon Spectral CT, Philips Medical Systems Netherland B.V.). Low radiation dose chest CT (64 x 0.625mm; voltage 120kV; current 70-140mA; gantry speed 270ms; pitch 1.23; slice thickness 2.0mm) was performed 5minutes after contrast injection. Since all patients with suspected AIS undergoing CT are quantitatively assessed online using automated software (RAPID, iSchemaView, Menlo Park, USA), a process that takes between 5 and 10minutes after contrast administration, delayed-enhancement spectral CT does not delay endovascular therapy.
All images were analyzed offline using dedicated software (IntelliSpace Portal version 11.1; Philips Medical Systems, Netherland B.V.) by a cardiovascular imaging expert with experience in dual energy cardiac CT blinded to the clinical history. Thrombi was defined as an abrupt focal filling defect with absent or nonsignificant contrast enhancement, clearly discriminated from surrounding structures such as blood and myocardial or vessel walls (figure 1).1,5 Regions of interest (ROI) were adjusted to the size of the thrombi, with special care taken to avoid adjacent structures. An ROI was placed at the ascending aorta, enabling the assessment of the thrombi/aortic ratio, with the lowest values reflecting the largest differences. Images were evaluated using conventional CT (Hounsfield units), monoenergetic imaging (Hounsfield units), at 40 keV, 55 keV, 70 keV, and 110 keV, and iodine-based results (mg/mL).
Patients with left atrial appendage (A), left ventricular (B), and aortic arch (C) thrombi evaluated using delayed-enhancement, low-dose, nongated, chest spectral computed tomography (CT) scans (delayed-enhancement spectral CT). Note that relatively vague differences using conventional CT (left panels) are more clearly discriminated by means of low monoenergetic (middle panels) and iodine density (right panels) imaging. Regions of interest (showing mean and standard deviation Hounsfield units [HU] and iodine densities) are deployed involving thrombi (arrows) and blood pool. Asterisks in panels B denote the presence of underlying myocardial infarction.
All participants provided written informed consent (habeas data) and this observational registry was approved by the institutional review board. The data supporting the findings of this study are available upon reasonable request.
From July 2020 to December 2021, 477 patients were admitted with an AIS. Of these, a cardiac or aortic thrombi was identified in 16 patients by using delayed-enhancement spectral CT. The mean age was 72.9±13.3 years, with a mean baseline National Institutes of Health Stroke Scale of 13.0±8.0. Six patients had atrial fibrillation and 6 had a history of coronary artery disease. All patients underwent transthoracic echocardiography during hospitalization, whereas 8 underwent advanced imaging with transesophageal echo or cardiac CT. Thrombi location comprised the left atrial appendage (LAA) in 6 patients, the left ventricle in 7 patients, and the aortic arch in 3 patients (figure 1). Although conventional CT yielded significant differences between the ascending aorta (blood) and thrombi (103.6±18.3 HU vs 44.7±13.7 HU; P<.0001), the largest differences were found using low monoenergetic imaging (40 keV=265.2±69.0 HU vs 69.0±32.7 HU, P<.0001; 55 keV=156.4±36.0 HU vs 53.3±19.2 HU, P<.0001; 70 keV=107.0±20.7 HU vs 46.3±13.7 HU, P<.0001; 110 keV=63.2±8.3 HU vs 40.0±10.0 HU, P<.0001) and iodine density maps (2.64±0.8mg/mL vs 0.38±0.3mg/mL; P<.0001) (table 1). The thrombi/aortic ratio were lower using iodine maps [conventional=0.44±0.16, 40 keV=0.27±0.13, 55 keV=0.36±0.14, 70 keV=0.44±0.16, 110 keV=0.65±0.19, iodine content=0.15±0.12, P (ANOVA)<.0001]. Compared with other locations, LAA thrombi showed the highest iodine content (left ventricle 0.21±0.18mg/mL vs LAA 0.89±0.62mg/mL vs aortic 0.18±0.14, mg/mL; P=.045).
Summary findings of density values (Hounsfield units), monoenergetic imaging (Hounsfield units) at increasing energy levels, and iodine-based results measured at the thrombotic source and at the ascending aorta
Conv (HU) | Monoenergetic (HU) | Iodine (mg/mL) | ANOVA | ||||
---|---|---|---|---|---|---|---|
40 keV | 55 keV | 70 keV | 110 keV | ||||
Aorta | 103.6 ± 18.3 | 265.2 ± 69.0 | 156.4 ± 36.0 | 107.0 ± 20.7 | 63.2 ± 8.3 | 2.64 ± 0.8 | |
Thrombi | 44.7 ± 13.7 | 69.0 ± 32.7 | 53.3 ± 19.2 | 46.3 ± 13.7 | 40.0 ± 10.0 | 0.38 ± 0.3 | |
P value | < .0001 | < .0001 | < .0001 | < .0001 | < .0001 | < .0001 | |
Thrombi/aorta | 0.44 ± 0.16 | 0.27 ± 0.13 | 0.36 ± 0.14 | 0.44 ± 0.16 | 0.65 ± 0.19 | 0.15 ± 0.12 | < .0001 |
Conv, conventional; HU, Hounsfield units; ANOVA, 1-way analysis of variance.
We demonstrated improved thrombi discrimination using spectral CT compared with conventional CT, with low energy monoenergetic imaging (40 keV) and iodine density maps showing the largest differences between thrombi and blood. Furthermore, we found variable iodine content according to the thrombus location and age, with LAA thrombi showing the highest iodine content compared with other locations. Accordingly, determination of single threshold values for the identification of thrombi might be inaccurate and should be specifically addressed in larger studies.6 The relatively small number of thrombi involved might lead to selection bias and influence comparative analysis according to location. In addition, given the reassuring images as shown, confirmation with advanced imaging was considered redundant in some patients.
AUTHORS’ CONTRIBUTIONSConception of the work, data analysis and interpretation, drafting, final approval, and assumption of responsibilities related to the content of the article: G.A. Rodríguez-Granillo. Design of the work, data collection, critical revision, final approval, and acceptance of responsibilities related to the content of the article: J. Cirio and Pedro Lylyk. Data collection, critical revision, final approval, and acceptance of responsibilities related to the content of the article: C. Bleise and L. Fontana.
FUNDINGNo funding.
AUTHORS’ CONTRIBUTIONSConception of the work, data analysis and interpretation, drafting, final approval, and assumption of responsibilities related to the content of the article: G.A. Rodríguez-Granillo. Design of the work, data collection, critical revision, final approval, and assumption of responsibilities related to the content of the article: J. Cirio and Pedro Lylyk. Data collection, critical revision, final approval, and assumption of responsibilities related to the content of the article: C. Bleise and L. Fontana.
CONFLICTS OF INTERESTNone of the authors has any conflicts of interest to declare related to the content of the manuscript.