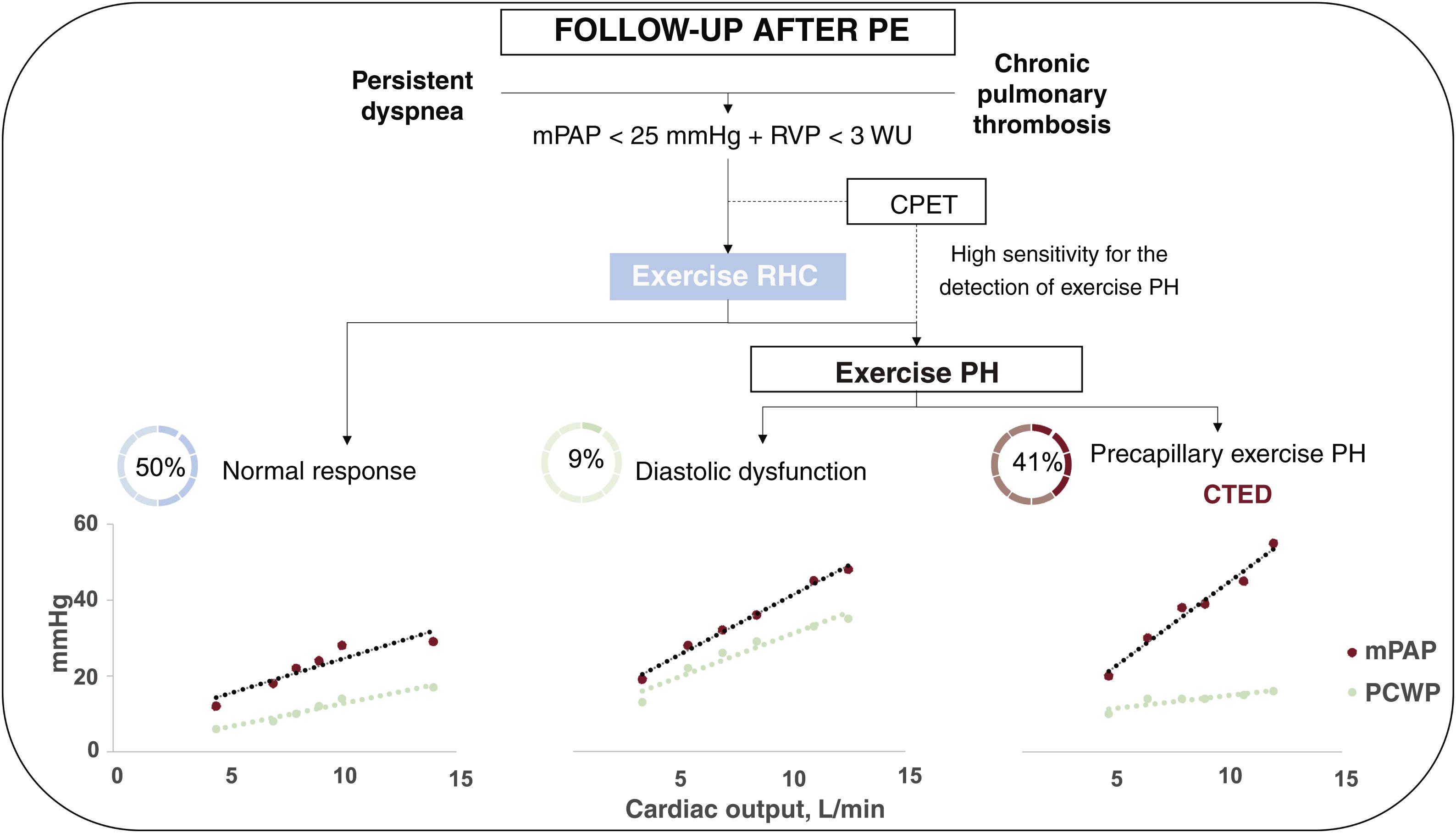
Chronic thromboembolic disease refers to the presence of chronic thrombotic pulmonary vascular thrombosis without pulmonary hypertension (PH) at rest but with exercise limitation after pulmonary embolism (PE). Our aim was to evaluate the hemodynamic response to exercise in these patients and its correlation with the values reached in cardiopulmonary exercise testing.
MethodsWe included symptomatic patients with persistent pulmonary thrombosis after PE. We excluded patients with left heart disease or significant PH (mean pulmonary arterial pressure [mPAP] >25mmHg, pulmonary vascular resistance >3 WU, and pulmonary capillary wedge pressure [PCWP] >15mmHg). Cardiopulmonary exercise testing and exercise right heart catheterization were performed. Exercise-induced precapillary PH was defined as mPAP/CO slope >3 and PCWP/CO slope <2mmHg/l/min. The hemodynamic response and the values obtained in cardiopulmonary exercise testing were compared between patients with and without exercise-induced precapillary PH.
ResultsWe studied 36 patients; 4 were excluded due to incomplete hemodynamic data. Out of the 32 patients analyzed; 3 developed a pathological increase in PCWP. Among the remaining 29 patients (mean age, 49.4±13.7 years, 34.5% women), 13 showed exercise-induced PH. Resting mPAP was higher in those who developed exercise-induced PH (23.3±5.4 vs 19.0±3.8mmHg; P=.012), although CO was similar in the 2 groups. Patients with exercise-induced PH exhibited data of ventilatory inefficiency with reduced values of end-tidal CO2 pressure at the anaerobic threshold (32.8±3.0 vs 36.2±3.3mmHg; P=.021) and a higher Ve/VCO2 slope (34.2±4.8 vs 30.7±5.0; P=.049).
ConclusionsExercise limitation and ventilatory inefficiency could be attributable to exercise-induced precapillary PH in a subgroup of patients with persistent pulmonary thrombosis and dyspnea.
Keywords
Chronic thromboembolic disease (CTED) is characterized by persistent thrombosis in the pulmonary vascular bed but no resting pulmonary hypertension (PH) after 1 or more episodes of symptomatic pulmonary embolism (PE) and correct anticoagulation for at least 3 months. It has been speculated that the functional limitations associated with CTED might be due to pulmonary microvasculopathy capable of causing exercise PH with dyspnea on exertion.1,2 The incidence and natural history of CTED are unknown.3
Patients with dyspnea that persists after 1 or more episodes of PE are considered to have post-PE syndrome. The prevalence of this syndrome has been estimated at 60% in follow-up series of patients with a history of PE.4,5 Incomplete thrombus resolution has been observed in 23% to 50% of series; in asymptomatic patients, it is normally an incidental finding and does not per se indicate a diagnosis of CTED.6 Chronic thromboembolic pulmonary hypertension (CTEPH), in turn, has been detected in 2% to 3% of patients 2 years after acute symptomatic PE,5 but this does not mean that all patients with a history of PE should be systematically screened for CTEPH. Structured follow-up has helped characterize and identify patients potentially at risk of CTEPH, and has also contributed to the definition of new entities with possible prognostic implications, such as CTED.5,7 Exercise hemodynamics are a useful tool in this setting, as detection of PH during exercise might indicate incipient vascular disease or chronic thromboembolic occlusions in the pulmonary vascular bed.8 The 2022 European Society of Cardiology/European Respiratory Society (ESC/ERS) guidelines define exercise PH as a mean pulmonary arterial pressure (mPAP)/cardiac output (CO) ratio of >3mmHg/L/min during exercise.9
Cardiopulmonary exercise testing (CPET) has become an important follow-up tool in the management of patients after PE.10,11 Ventilatory inefficiency, understood as an increasing need for ventilation to achieve effective gas exchange, is a hallmark of CTEPH (but not other forms of PH), and should raise alarms if detected during follow-up of PE.6,12
We hypothesize that functional limitations in patients with persistent thrombosis after PE can be explained by an impaired hemodynamic response to exercise that can be detected by exercise right heart catheterization (RHC) and predicted using noninvasive techniques such as CPET. The aim of our study was to analyze exercise-induced hemodynamic changes in a cohort of patients with dyspnea and persistent thrombosis after PE and to explore associations with CPET variables.
METHODSPatientsObservational study of a cohort of patients aged >18 years who were referred to a PH unit with a suspected diagnosis of CTEPH during follow-up after PE. The inclusion criteria were a) presence of symptoms (functional class ≥II); b) persistent perfusion defects on ventilation/perfusion scintigraphy and images of chronic thromboembolic occlusions in the pulmonary vascular bed after 3 months of correct anticoagulation; c) baseline hemodynamics showing an absence of significant PH, including patients with a mean pulmonary artery pressure (mPAP) <25mmHg, pulmonary vascular resistance (PVR) <3 Wood units (WU), and pulmonary capillary wedge pressure (PCWP) ≤15mmHg; d) absence of left heart disease; e) absence of lung disease (table 1 of the supplementary data); and f) informed consent to participate in the study. The study protocol was approved by the hospital's ethics committee.
Study designPatients were included in the study after an initial clinical evaluation. Ventilation/perfusion scintigraphy and computed tomography pulmonary angiography were performed using standard protocols at our hospital.13 Left heart disease was ruled out in all patients by clinical assessment and echocardiography (EPIQ CVx, Philips Healthcare, USA).
CPET was performed on a cycle ergometer with continuous gas collection and heart monitoring (Blue Cherry Ergoline, Sanro, Spain). Lung function tests were performed just before CPET to rule out obstructive or restrictive disease. An incremental CPET protocol consisting of a baseline work rate of 20 W with 5-W increments every 30 seconds was used. The variables measured were ventilation (Ve), oxygen (O2) uptake and carbon dioxide (CO2) production. Variables extracted for analysis were peak O2 uptake (VO2); ventilatory equivalents for CO2 and O2; Ve/VCO2 slope; partial pressures of end-tidal CO2 (PetCO2) and O2 (PetO2) at rest, anaerobic threshold (AT), and peak exercise; O2 uptake efficiency slope; and respiratory exchange ratio (RER). Heart rate, systemic blood pressure, continuous electrocardiographic tracings, and noninvasive peripheral O2 saturation were recorded for all patients.
Within 24 hours of the CPET, the same protocol was used to perform supine exercise RHC on a cycle ergometer in the catheterization laboratory. A 7-Fr sheath and Swan-Ganz catheter (Edwards Lifesciences, USA) were inserted via peripheral venous access to obtain baseline hemodynamics with measurement of pressures. Pulmonary pressure was monitored continuously during the exercise protocol. Baseline CO was estimated using the thermodilution technique, with calculation of the mean of at least 3 measurements. For each work rate increment, at least 1 measurement was obtained for CO, PCWP and mixed venous O2 saturation. PCWP was measured at end expiration both at rest and during exercise and averaged over 10 seconds. The hemodynamic variables calculated were PVR (mPAP–PCWP)/CO and vascular compliance (stroke volume/pulse pressure). At least 3 mPAP, PCWP, and CO measurements during exercise were required to consider the study valid. Hemodynamic responses were classified as abnormal when the mPAP/CO slope exceeded 3mmHg/L/min. A PCWP/CO ratio >2mmHg/L/min was used to define heart failure with preserved ejection fraction (HFpEF).9,14 A subanalysis of hemodynamic responses was performed according to the presence or absence of PH at rest, defined using the new hemodynamic criterion in the latest ESC/ERS guidelines on PH: mPAP >20mmHg and PVR >2 WU.9
Statistical analysisStatistical analyses were performed in Stata version 17.0 (Stata Corp., USA). Continuous variables were compared using the t test or the Wilcoxon test depending on the normality of distribution, while categorical variables were compared using the chi-square test. The pressure-volume relationship was estimated individually by linear regression analysis of the points obtained during exercise (mPAP/CO and PCWP/CO). Correlations between continuous variables were analyzed using Pearson correlation coefficients. Receiver operating characteristic curves were built to determine maximum sensitivity and specificity and the area under the curve (AUC) for each CPET variable in order to identify impaired hemodynamic responses to exercise. Statistical significance was set at a P value of less than .05.
RESULTSBaseline characteristics of the study populationFour of the 36 patients initially selected were excluded due to poor-quality hemodynamic measurements. The CPET studies in the remaining 32 patients (88.8%) were considered valid. Sixteen patients (50%) developed exercise PH. In 3 of these patients, the exercise PH was postcapillary and uncovered HFpEF (figure 1). All subsequent analyses focused on the other 29 patients to compare patients with precapillary exercise PH (CTED) and those with a normal hemodynamic response to exercise. There were no complications. The baseline characteristics of the 29 patients are summarized in table 1. No differences were observed between the groups for cardiovascular risk profile, age, or sex. Median time from PE to hemodynamic testing was similar in both groups (3.4 [2.4-4.1] vs 2.3 [1.7-4.3] years, P=.376). All patients were in New York Heart Association functional class II, and none of them were receiving treatment with pulmonary vasodilators. The baseline echocardiograms showed no evidence of left ventricular diastolic dysfunction in either group (table 2 of the supplementary data).
Baseline characteristics of patients overall and stratified by hemodynamic response to exercise
All patients (n=29) | Hemodynamic response | P | ||
---|---|---|---|---|
Abnormal (n=13) | Normal (n=16) | |||
Age, y | 49.4±13.7 | 49.6±16.3 | 49.1±11.7 | .803 |
Women | 10 (34.5) | 4 (31.0) | 6 (37.5) | .507 |
BMI | 25.6 [23.8-29.7] | 25.7 [24.5-29.7] | 25.2 [23.2-29.9] | .746 |
Years since PE | 3.1 [1.9-4.1] | 3.4 [2.4-4.1] | 2.3 [1.7-4.3] | .376 |
PE risk | ||||
Intermediate-high | 14 (48.3) | 7 (69.2) | 5 (31.3) | .132 |
High | 4 (13.8) | 1 (7.7) | 3 (18.8) | |
Fibrinolysis | 3 (10.2) | 0 | 3 (18.8) | .153 |
Hypertension | 9 (31.0) | 5 (38.5) | 4 (25.0) | .353 |
Diabetes mellitus | 3 (10.2) | 2 (15.4) | 1 (6.3) | .420 |
Former smoker | 6 (20.7) | 2 (15.4) | 4 (25.0) | .435 |
LVEF, % | 58.7±3.3 | 58.6±3.7 | 59.0±3.2 | .802 |
Tei index | 0.32 [0.29-0.46] | 0.38 [0.36-0.46] | 0.30 [0.28-0.32] | .160 |
S’, cm/s | 12.1 [11.5-13.0] | 12.0 [11.5-12.3] | 12.7 [12.0-13.5] | .182 |
TAPSE, mm | 21.1±3.4 | 19.4±2.7 | 22.3±3.4 | .052 |
RVFWS, % | –22.0±3.3 | –21.2±3.2 | –22.7±3.7 | .889 |
PASP, mmHg | 29.8±6.0 | 30.7±8.9 | 29.2±4.9 | .618 |
BMI, body mass index; LVEF, left ventricular ejection fraction; PASP, pulmonary artery systolic pressure; PE, pulmonary embolism; RVFWLS, right ventricular free wall longitudinal strain.
Values are expressed as No. (%), mean±standard deviation, or median [interquartile range].
Thirteen patients showed an abnormal hemodynamic response to exercise (4.21±1.5 vs 1.94±0.6mmHg/L/min) (figure 2A). The hemodynamic variables at rest and peak exercise are shown in table 2. Patients with an impaired response had higher mPAP (23.3±5.4 vs 19.0±3.8mmHg, P =.012) and PVR at rest (2.1±0.9 vs 1.2±0.6WU, P=.005), with similar CO (5.7± 1.3 vs 5.7±1.1 L/min, P=.957). Mean PCWP was similar in both groups at rest (11.6±2.5 vs 12.0±2. 7mmHg, P=.653), but was higher at peak exercise in patients with an abnormal hemodynamic response to exercise (52.4±9.7 vs 34.7±5.7mmHg, P<.001). The groups had similar values for mean peak CO (12.6±2.7 vs 13.4±1.9 L/min, P=.365) and mean PCWP at maximal exercise (22 .0±4.7 vs 21.2±4.7mmHg, P=.566). Differences in PCWP/CO ratio were also nonsignificant (1.40±0.4 vs 1.18±0.4mmHg/L/min, P =.568) (figure 2B).
Hemodynamic responses to exercise in patients with chronic thromboembolic disease. A: mean mPAP/CO slope according to hemodynamic response; B: PCWP/cardiac output slope according to hemodynamic response. mPAP, mean pulmonary arterial pressure; PCWP, pulmonary capillary wedge pressure.
Hemodynamic response at baseline and at peak exercise according to hemodynamic response
All patients (n=29) | Hemodynamic response | P | ||
---|---|---|---|---|
Abnormal (n=13) | Normal (n=16) | |||
Hemodynamics at rest | ||||
RV, mmHg | 6.8±2.6 | 6.4±2.5 | 7.1±2.7 | .388 |
mPAP, mm Hg | 20.9±5.1 | 23.3±5.4 | 19.0±3.8 | .012 |
CO, L/min | 5.7±1.2 | 5.7±1.3 | 5.7±1.1 | .957 |
TVR, WU | 3.8±1.0 | 4.2±0.9 | 3.4±1.0 | .029 |
PCWP, mmHg | 11.8±2.5 | 11.6±2.4 | 12.0±2.7 | .653 |
RVP, WU | 1.6±0.8 | 2.1±0.9 | 1.2±0.6 | .005 |
Compliance, mL/mmHg) | 4.7±2.1 | 3.4±0.9 | 5.7±2.2 | .001 |
Criterion for PH at rest (2022) | ||||
mPAP >20 mmHg+RVP >2 UW | 8 (27.6) | 7 (53.8) | 1 (6.3) | .008 |
Hemodynamics at peak exercise | ||||
mPAP, mm Hg | 42.7±11.8 | 52.4±9.7 | 34.7±5.7 | <.001 |
CO, L/min | 13.1±2.3 | 12.6±2.7 | 13.4±1.9 | .365 |
TVR, WU | 3.3±1.1 | 4.3±0.9 | 2.6±0.5 | <.001 |
PCWP, mmHg | 21.5±4.6 | 22.0±4.7 | 21.2±4.7 | .566 |
RVP, WU | 1.6±1.0 | 2.6±0.9 | 1.0±0.4 | <.001 |
Compliance, mL/mmHg) | 3.5±2.2 | 1.8±0.6 | 4.9±2.0 | <.001 |
Work rate, W | 109.8±36.0 | 110.8±41.8 | 109.1±32.0 | .922 |
Slopes | ||||
mPAP/CO, mmHg/L/min | 2. 92±1.5 | 4.21±1.5 | 1.94±0.6 | <.001 |
PCWP/CO, mmHg/L/min | 1.27±0.5 | 1.40±0.4 | 1.18±0.4 | .568 |
Measurement points | 5 [4-6] | 6 [5-8] | 5 [4-6] | .111 |
CO, cardiac output; mPAP, mean pulmonary arterial pressure; PCWP, pulmonary capillary wedge pressure; PH, pulmonary hypertension; PVR, pulmonary vascular resistance; RV, right ventricle; TVR, total vascular resistance.
Values are expressed as No. (%), mean±standard deviation, or median [interquartile range].
PVR and vascular compliance at rest were both correlated with the mPAP/CO slope (r=0.654, P=.001 and r=–0.529, P=.008 respectively). No correlation was observed between this slope and mPAP at rest (r=0.333, P=.083).
Eight patients (27.6%) met the new hemodynamic criterion for PH at rest (mPAP >20mmHg and PVR >2 WU). Of these, 7 (88%) developed exercise PH.
CPET and hemodynamic response to exerciseThe CPET results are shown in table 3. Patients with abnormal and normal hemodynamic responses to exercise reached a similar work rate (127.3±55.2 vs 126.8±27.6 W, P=.759) and had a similar mean peak RER during maximal exercise (1.15±0.6 vs 1.16±0.1, P=.992). There were no differences in blood pressure, heart rate, or peripheral O2 saturation during exercise (table 3 of the supplementary data). Differences in peak VO2 were nonsignificant, with slightly lower-than-predicted values in both groups (73.4%±20.1% vs 79.7%±13.2%, P=.558).
Cardiopulmonary exercise test results overall and stratified by hemodynamic response
All patients (n=29) | Hemodynamic response | |||
---|---|---|---|---|
Abnormal (n=13) | Normal (n=16) | P | ||
Cardiovascular response | ||||
RER at peak exercise | 1.15±0.7 | 1.15±0.6 | 1.16±0.1 | .992 |
VO2 at peak exercise, mL/min/kg | 21.2±6.3 | 20.9±7.6 | 21.4±5.2 | .770 |
VO2 at peak exercise, % predicted | 76.8±16.7 | 73.4±20.1 | 79.7±13.2 | .558 |
VO2 at AT, mL/min/kg | 14.1±4.4 | 13.9±5.3 | 14.3±3.6 | .888 |
VO2 at AT, % predicted | 49.9±15.7 | 45.2±18.5 | 53.6±12.3 | .288 |
Oxygen pulse, mL/beat | 11.4±15.7 | 11.0±3.4 | 11.7±2.9 | .777 |
O2 pulse, % predicted | 87.3±17 | 81.2±15.4 | 92.5±16.9 | .188 |
OUES, % predicted | 76.0±27.7 | 67.6±34.1 | 82.6±20.1 | .622 |
Ventilatory efficiency | ||||
PetCO2 at rest, mmHg | 30.9±2.8 | 30.0±2.9 | 31.8±2.6 | .106 |
PetCO2 at AT, mmHg | 34.7±3.5 | 32.8±3.0 | 36.2±3.3 | .021 |
PetCO2 at peak exercise, mmHg | 31.8±3.4 | 30.8±3.4 | 32.7±3.4 | .222 |
Equivalent for CO2 at AT, mmHg | 31.9±3.6 | 33.2±3.7 | 30.9±3.1 | .134 |
Equivalent for O2 at peak exercise, mmHg | 39.3±5.4 | 39.2±6.0 | 39.3±5.1 | .698 |
Ve/CO2 slope | 32.4±5.2 | 34.2±4.8 | 30.7±5.0 | .049 |
Ventilation | ||||
Breathing reserve, % | 61.3±17.5 | 57.0±22.5 | 64.9±11.3 | .533 |
Work load | ||||
Work rate, W | 127.0±42.1 | 127.3±55.2 | 126.8±27.6 | .759 |
AT, anaerobic threshold; O2, oxygen; PetCO2, partial pressures of end-tidal carbon dioxide; RER, respiratory exchange rate; Ve, pulmonary ventilation; VO2, oxygen uptake.
Values are expressed as No. (%) or mean±standard deviation.
In the ventilatory efficiency assessment, PetCO2 at AT was significantly lower in patients who developed exercise PH (32.8±3.0 vs 36.2±3.3mmHg, P=.021). These patients also had a steeper Ve/CO2 slope (34.2±4.8 vs 30.7±5.0, P=.049)
PetCO2 at AT and the Ve/CO2 slope were moderately correlated with the mPAP/CO slope (r=-0.544, P=.002 and r=0.594, P<.001, respectively). The AUC values indicating the discriminatory ability of the ventilatory efficiency variables to predict exercise PH were 0.750 (95%CI, 0.567-0.933) for PetCO2 at AT and 0.744 (95%CI, 0.513-0.868) for the Ve/VCO2 slope (figure 3). A PetCO2 value <33mmHg at AT had a sensitivity of 100% and a specificity of 46.5% for the detection of exercise PH with a negative predictive value of 100%. The corresponding values for a mean Ve/VCO2 slope >31.5 was a sensitivity of 80.4%, a specificity of 50.0%, and a negative predictive value of 80.0%.
DiscussionThis is one of the largest cohorts to study the use of exercise RHC in the diagnostic evaluation of CTEPH. The main findings are a) 40.6% of patients with suspected CTEPH without PH at rest had an impaired hemodynamic response to exercise indicative of pulmonary vasculopathy, b) 88% of patients with mPAP of 20 to 25mmHg and PVR of 2 to 3 WU developed exercise PH, c) exercise RHC is a useful test for uncovering HFpEF as a cause of dyspnea, and d) ventilatory inefficiency during CPET is associated with exercise PH.
PE, with an incidence of 48 to 115 cases per 100 000 inhabitants, is the third leading cause of cardiovascular admissions, behind acute myocardial infarction and stroke.15 Structured follow-up of patients with a history of PE led to the formulation of a new concept known as post-PE syndrome, which covers different conditions ranging from asymptomatic persistent pulmonary thrombosis to CTEPH.4 The high proportion of patients with functional limitations or persistent dyspnea after acute PE, however, contrasts with the low incidence of CTEPH in prospective cohorts.5 CTEPH is characterized by vascular occlusions, which if left untreated, are associated with similar mortality to that of untreated group 1 PH.16 Concern about the magnitude of this problem among scientific societies is reflected in the creation of PE follow-up algorithms. The 2019 ESC guidelines, for example, recommend a multidisciplinary approach.17 Muscle weakness and cardiovascular comorbidities appear to play an important role in persistent dyspnea after PE, but they do not explain the functional limitations observed in other patients, largely, as in our study, young adults without comorbidities. Our cohort comprised middle-aged patients (49.4±13.7 years) with a deteriorated functional class or de novo dyspnea after PE who had persistent pulmonary artery thrombosis without PH at rest and no other causes of dyspnea. It has been speculated that residual thrombosis in CTED may lead to vascular dysfunction under stress conditions, manifested by an abnormal hemodynamic response, but it is unknown whether this a precursor to CTEPH.1,2,9
Exercise RHC produced 3 types of hemodynamic responses in our patients: a) precapillary exercise PH in 13 patients, b) postcapillary exercise PH in 3 patients, and c) a normal hemodynamic response in 16 patients. An abnormal precapillary response is indicative of vascular disease or residual thromboembolic material, that is, CTED. Compared with patients with a normal hemodynamic response to exercise, those with an abnormal precapillary response had higher mPAP at rest and a disproportionate increase in this variable during exercise, despite a similar increase in CO. Delcroix and colleagues, in a comprehensive cardiopulmonary assessment of patients with CTED and CTEPH, analyzed right ventricular volume changes using exercise cardiac magnetic resonance imaging and simultaneous invasive pressure measurements.18 The 14 patients with CTED had a similar profile to our cohort and their response to exercise was characterized by a disproportionate increase in afterload, reflected in a median mPAP/CO slope of 3.5 [2.2-4.4] mmHg/L/min. This response, in turn, was associated with an insufficient reduction in expected right ventricular end-systolic volume compared with a group of 13 healthy adults, indicating a reduction in right ventricular ejection fraction. These findings suggest that CTED represents an intermediate phenotype with respect to CTEPH, characterized by right ventricular dilation at rest and absence of contractile reserve, preventing an increase in output during exercise.
Surgical removal of thromboembolic material in symptomatic patients without PH at rest has been associated with clinical and hemodynamic benefits. Taboada et al.,19 the first authors to report on the use of thromboendarterectomy in patients with CTED, reported improved functional class in 95% of the 42 patients studied. Nonetheless, 40% of patients experienced complications, although there were no in-hospital deaths. Our group reported similar findings for patients with CTED without PH at rest who underwent the same procedure.20 Guth et al.21 showed that hemodynamic responses to exercise were restored to normal in a cohort of 12 patients with CTED who underwent pulmonary thromboendarterectomy (mean mPAP/CO ratio of 3.6±1.0mmHg/L/min before the intervention vs 2.3±0.8mmHg/l/min afterwards, P=.002). The patient profiles in the above series were similar: a mean age of 35 to 55 years, absence of comorbidities, surgically accessible proximal bilateral chronic thrombotic material, mPAP at rest of 20 to 25mmHg, and PVR of 1.8 to 3 WU. Pulmonary angioplasty for CTED has attracted interest due to its low complication rates and similar clinical outcomes to those observed with surgery.22,23 In line with the above findings, the 2002 ESC/ERS guidelines for the diagnosis and treatment of PH consider that pulmonary endarterectomy should be contemplated in selected patients with CTED in whom other causes of dyspnea have been ruled out (class IIa recommendation, level C evidence).9
CPET has gained popularity as an option for all groups of patients with PH in recent years, but its usefulness has traditionally been limited by the difficulty of establishing a threshold determining pathologic changes owing to variations in age, sex, and comorbidities across the studies.14 The recent ESC/ERS guidelines defined the criterion for exercise PH and reduced the thresholds for precapillary PH at rest to mPAP >20mmHg, PVR >2 WU, and PCWP ≤ 15mmHg.9 This new definition does not mean that patients exceeding these thresholds must necessarily be treated. The recommendation for patients with suspected CTED is to carefully rule out other potential causes of dyspnea by analyzing impaired responses to exercise (exercise PH) and checking for HFpEF. Our study sheds light on a group of patients previously considered to be in a gray zone and supports the recent guideline changes, as 8 of the patients studied met the new hemodynamic criterion for PH at rest. Within this group, all but 1 patient developed exercise PH. Of the 21 patients with normal resting hemodynamics (mPAP <20mmHg and PVR <2WU), 6 developed precapillary exercise PH. In our experience, thus, the current hemodynamic criterion identifies most patients who develop PH during exercise, although we also observed a subgroup of CTED patients with normal mPAP at rest but an abnormal hemodynamic response to exercise.
In our opinion, exercise RHC is a potentially useful tool for selecting which of the above patients could benefit from pulmonary endarterectomy. It is a safe diagnostic technique with similar complication rates to those observed for RHC without exercise.14 No complications occurred in our series. Furthermore, exercise RHC uncovered HFpEF as the origin of dyspnea on exertion in 3 patients, leading to reconsideration of the underlying cause based on the finding of a PCWP/CO ratio during exercise >2mmHg/L/ min, which in addition, had diagnostic and prognostic value.24 These considerations suggest that exercise RHC might be even more useful in the study of symptomatic patients with suspected CTED without PH at rest, as 9% of the patients in our series had postcapillary exercise PH, despite no evidence of this in the echocardiogram and normal left ventricular filling pressures (figure 4).
Central illustration. Hemodynamic results in exercise RHC in patients with suspected CTED. CPET, cardiopulmonary exercise testing; CTED, chronic thromboembolic disease; mPAP, mean pulmonary arterial pressure; PCWP, pulmonary capillary wedge pressure; PH, pulmonary hypertension; PE, pulmonary embolism; PVR, pulmonary vascular resistance; RHC, right heart catheterization.
CPET is also a very useful noninvasive test for characterizing dyspnea in multiple conditions. In our cohort, patients with normal and abnormal hemodynamic responses to exercise had slightly reduced peak VO2 values, similar to reports in other PE follow-up series.25 Those who developed exercise PH, however, showed altered ventilatory efficiency variables (PetCO2, VCO2, Ve/VCO2 slope), especially at AT. The degree of ventilatory inefficiency appears to be associated with hemodynamic status in CTEPH, and patients with CTED appear to have an intermediate phenotype, between that of healthy patients and patients with CTEPH at rest.18 Notably, we observed fewer alterations to mean Ve/CO2 and PetCO2 values compared with other studies of CTED,26 possibly because the patients in our series were on average younger and because we excluded patients with findings indicative of HFpEF during exercise. Despite our small sample, PetCO2 kinetics, especially when analyzed at AT, and the Ve/VCO2 ratio derived from PCWP had reasonable discriminatory ability to identify patients who would subsequently develop exercise PH. Based on our findings, which require external validation in a larger number of patients, it can be concluded that patients with normal CPET results during PE follow-up will not develop exercise PH. We therefore propose using CPET as a screening tool to investigate the causes of dyspnea before invasive tests, such as exercise RHC.
LimitationsThis was a small, single-center, cross-sectional study showing an association between exercise PH and ventilatory inefficiency in CPET. Nonetheless, it cannot be concluded that persistent dyspnea is caused by either of these conditions, as no clinical follow-up studies were performed.
The patients are not fully representative of patients with post-PE syndrome, as they had a low cardiovascular risk profile and no comorbidities. They were referred to the PH unit at our hospital with suspected CTEDPH to evaluate possible treatments for persistent symptoms, possibly explaining the higher prevalence of exercise PH observed.
The new definition of PH at rest applied to this study influenced our inclusion criteria. However, because both evidence and recommendations for the treatment of mild PH according to current hemodynamic criteria are lacking, we also accepted patients with mPAP of 20 to 24mmHg and PVR of 2 WU and performed relevant subanalyses.
CONCLUSIONSPrecapillary exercise PH was associated with functional limitations in patients who had persistent dyspnea after PE and chronic pulmonary vascular bed thrombosis and no other causes that could explain their symptoms. CPET is a useful technique for exploring persistent idiopathic dyspnea, as it discriminates between precapillary exercise PH, potentially indicating underlying pulmonary microvasculopathy, and postcapillary exercise PH typically associated with HFpEF. CPET, in turn, is a noninvasive test capable of predicting exercise PH.
- –
Assessment of hemodynamic response to exercise is useful for studying dyspnea on exertion in patients without PH.
- –
Functional limitations after PE are common, have diverse causes, and require multidisciplinary treatment.
- –
Most cases of CTED without PH are diagnosed during structured follow-up after PE.
- –
Exercise PH, diagnosed by RHC performed during exercise, occurs in a subgroup of patients with functional limitations after PE.
- –
Identification of these patients could have prognostic implications, as they could benefit from early invasive or pharmacologic treatment that could improve their prognosis and quality of life.
- –
Ventilatory inefficiency in CPET is a valuable noninvasive diagnostic metric for patients with exercise PH.
This project is supported by research grants received from the Spanish Society of Cardiology in 2019. Alejandro Cruz-Utrilla was awarded the Río Hortega grant by the Instituto de Salud Carlos III of the Spanish Ministry of Science and Innovation (CM20/00164).
AUTHORS’ CONTRIBUTIONSS. Huertas, M. Velázquez, and P. Escribano conceived and designed the project. S. Huertas, M. Velázquez, F. Sarnago, N. Maneiro, C. Jiménez, and T. Segura de la Cal contributed to patient selection, performance of invasive tests, and data analysis.
A. Flox, T.A. Cruz, R. Aguilar, M.J. López, C. Andrés, Y. Revilla, S. Alonso, C. Gómez, and F. Arribas contributed to patient selection and follow-up. All the authors contributed to writing and critically reviewing this manuscript. They also all approved the final version and are responsible for its content.
CONFLICTS OF INTERESTThe authors declare that they have no conflicts of interest.