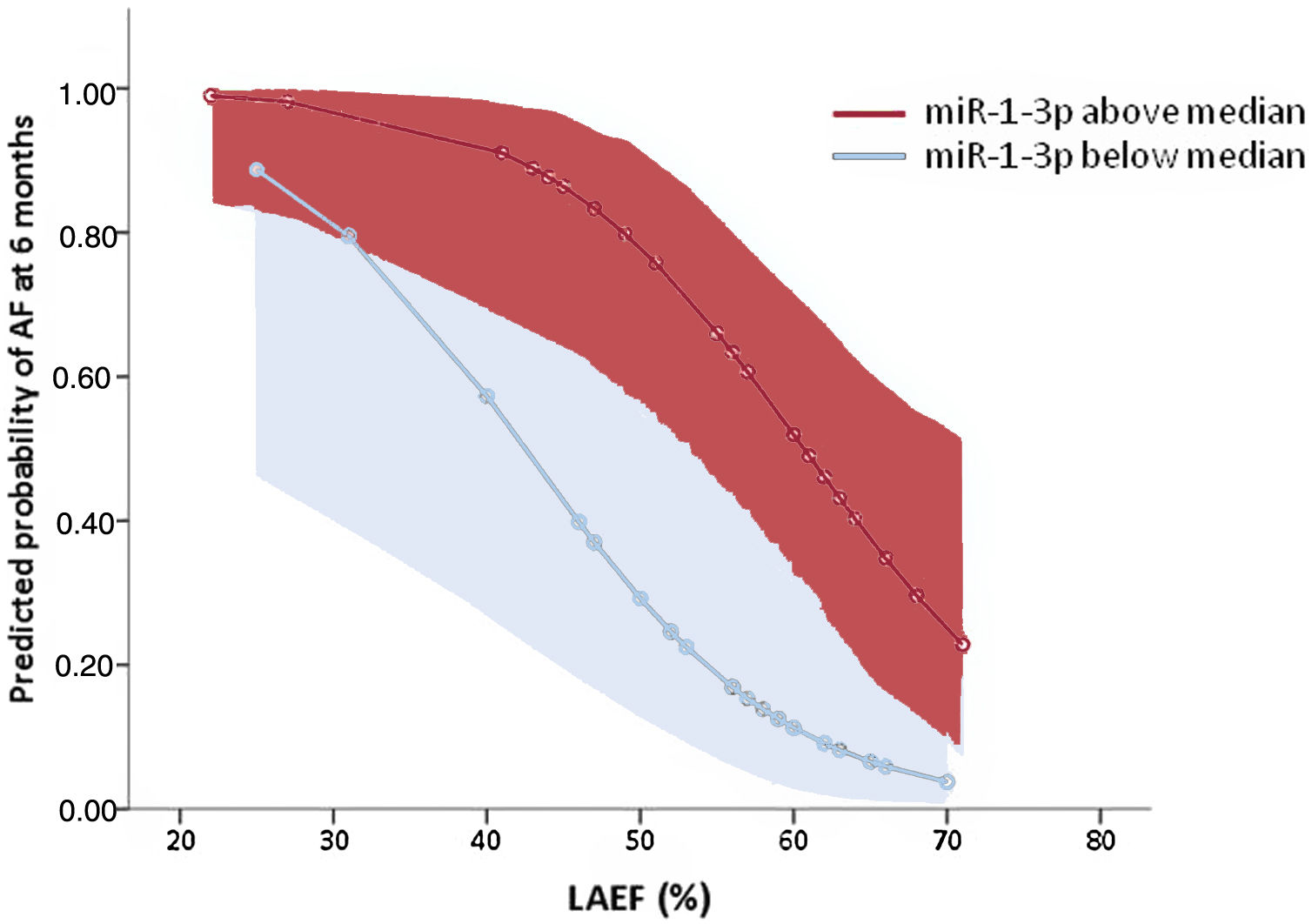
Identifying biomarkers of subclinical atrial fibrillation (AF) is of most interest in patients with cryptogenic stroke (CrS). We sought to evaluate the circulating microRNA (miRNA) profile of patients with CrS and AF compared with those in persistent sinus rhythm.
MethodsAmong 64 consecutive patients with CrS under continuous monitoring by a predischarge insertable monitor, 18 patients (9 with AF and 9 in persistent sinus rhythm) were selected for high-throughput determination of 754 miRNAs. Nine patients with concomitant stroke and AF were also screened to improve the yield of miRNA selection. Differentially expressed miRNAs were replicated in an independent cohort (n=46). Biological markers were stratified by the median and included in logistic regression analyses to evaluate their association with AF at 6 and 12 months.
ResultsEight miRNAs were differentially expressed between patients with and without AF. In the replication cohort, miR-1-3p, a gene regulator involved in cardiac arrhythmogenesis, was the only miRNA to remain significantly higher in patients with CrS and AF vs those in sinus rhythm and showed a modest association with AF burden. High (= above the median) miR-1-3p plasma values, together with a low left atrial ejection fraction, were independently associated with the presence of AF at 6 and 12 months.
ConclusionsIn this cohort, plasma levels of miR-1-3p were elevated in CrS patients with subsequent AF. Our results preliminarily suggest that miR-1-3p could be a novel biomarker that, together with clinical parameters, could help identify patients with CrS and a high risk of occult AF.
Keywords
Around 30% of ischemic strokes are of undetermined etiology and therefore classified as cryptogenic (CrS).1,2 Previous works have suggested that a significant proportion of CrS may be secondary to occult paroxysmal atrial fibrillation (AF), which can be subsequently documented in follow-up.1,3 The best strategy for AF detection in CrS patients, however, is poorly established.3 Subcutaneous insertable cardiac monitors, allowing for continuous 24/7 heart rhythm monitoring, seem to be the most attractive alternative,3–6 but their cost-effectiveness is yet to be established. More importantly, their diagnostic yield relies on the identification of an episode of AF, which may occur weeks or months after the index stroke, leaving patients at potential risk undertreated until AF is documented.
A more desirable approach would therefore be to identify indirect markers of subclinical AF that could be detected at the moment of first admission. AF has a complex pathophysiology, in which ectopic activity over a particular predisposing atrial remodeling converges to trigger and sustain the arrhythmia.7 It is reasonable to speculate that a predisposing environment facilitating such mechanisms would exist in patients with CrS with underlying occult AF, which could be potentially detected by means of biological markers. In recent years, microRNAs (miRNAs), small RNA sequences that regulate gene expression, have been directly involved in the pathophysiology of AF.8 Additionally, because a good tissue-blood correlation has been described, these molecules have been proposed as potential biological markers of the arrhythmia.9,10 Preliminary data have been published on the miRNA signatures present in the blood of individuals with a future incident AF, and in patients with some particular forms of persistent and long-standing AF.11–14 Less information is available on the circulating miRNA profile of patients with paroxysmal AF, which mostly comes from reports focused on specific miRNAs rather than high-throughput screenings.15
To date, there is no information on the circulating miRNA profile in patients with CrS and potentially occult AF. Therefore, in this study, we sought to describe the differential miRNA expression in patients with CrS and subclinical AF compared with those in persistent sinus rhythm (SR), under the hypothesis that the former would present a particular circulating miRNA profile predisposing to the arrhythmia.
METHODSStudy population and standard of careIndividuals were selected consecutively from a prospective cohort of patients with CrS admitted to the stroke unit of Hospital del Mar (Barcelona, Spain) between October 2013 and September 2016 and meeting the following criteria6: a) ischemic stroke or transient ischemic attack; b) age between 50 and 89 years; c) undetermined origin according to the SSS-TOAST criteria.2 We excluded patients with a history of hemorrhagic stroke, prior AF or atrial flutter, permanent contraindication for oral anticoagulation, recent (< 1 month) major surgery or cardiac events, severe cardiac disease or comorbidities, short life expectancy (< 1 year), or severe stroke (modified Rankin Scale >4).6 The study followed national and international principles (Declaration of Helsinki) and was approved by the local ethics committee (2013/5055/I). All patients or next-of-kin were required to sign the specific informed consent form.
Blood was obtained from a peripheral line at the time of admission. Patients were immediately treated with antiplatelet therapy and transferred to the stroke unit under continuous electrocardiogram-monitoring. A 2-dimensional transthoracic echocardiogram was performed within the first 48hours with a General Electric Vivid E9 equipment (GE Healthcare, Chicago, IL, USA) to assess the dimensions and functionality of the left ventricle and left atrium. See further details in the .
As per protocol, all patients with no established cause for the stroke underwent implantation of an insertable cardiac monitor when transferred to the general ward. Insertable cardiac monitor devices (Biomonitor and Biomonitor-2, Biotronik, Berlin, Germany) were programmed with the specific algorithm for AF detection, defined by an RR interval variability> 12.5% lasting> 1minute, as previously described.6 Recordings automatically detected as potential AF episodes were reviewed by a specialized cardiologist. AF burden was assessed by the percentage of monitoring time an individual was in AF, and secondarily by the number of AF episodes, as previously suggested.16 An AF time <0.05% defined participants with low AF burden, whereas AF burden was high when AF time was ≥ 0.05%.17 If AF was documented at any time, oral anticoagulation was initiated.
Study groups and designThis was a case-control study in which patients were prospectively enrolled and allocated to cases (patients with AF, CrS-AF) or controls (patients in continuous SR, CrS-SR) after 6 months of monitoring, the final timepoint of the primary analysis. A secondary analysis was performed at 12 months of follow-up.
As commonly performed in gene expression studies,18 the study design followed a 2-step approach, including a discovery phase using high-throughput microarray data, with selection of potential candidate biomarkers, followed by a replication (and confirmatory) phase of candidate miRNAs in an independent cohort (figure 1). For the discovery phase, a total of 18 age- and sex-matched patients were selected (set I): 9 CrS-AF and 9 CrS-SR. All 9 patients in the 2 groups were followed up for 12 months to ensure that they remained equally classified either at 6 or 12 months (ie, no AF episodes occurred in the CrS-SR beyond 6 months), validating their eligibility for the study at 6 and 12 months. In parallel, an additional group of 9 patients (with no prior history of AF) presenting with AF at admission (cardioembolic stroke, CES-AF), also matched for the previous groups, was screened in this phase with the aim of further guiding the selection of miRNAs. For the replication phase, we used an independent cohort of 46 unmatched patients (set II), of which 19 had AF at 6 months. The same cohort was analyzed after 12 months of follow-up, when 23 had shown AF (figure 1).
MicroRNA extraction and profilingMiRNAs were isolated from plasma using the miRNeasy Serum/Plasma kit (Qiagen Hilden, Germany). A TaqMan OpenArray Human Advanced MicroRNA Panel (ThermoFisher, Waltham, MA, USA) analyzing 754 human miRNAs was used in the discovery phase, and expression levels of all miRNAs were analyzed with the Cloud software (ThermoFisher). Those miRNAs found to have a significant differential expression among groups (P <.05 and fold change> 2 or <.5) were selected for replication, which was performed by quantitative polymerase chain reaction (qPCR). The results were analyzed using the Expression Suite software (Life Technologies). See further details in the .
Statistical analysisClinical data are reported as mean±standard deviation or frequency (%). MiRNA expression results are expressed in fold change vs controls, where 1 equals the mean of expression of all controls and therefore represents the reference value.19 OpenArray analysis of differential expression was assessed with the specific Cloud Software (Life Technologies) using the global normalization method. Other comparisons were performed with the Student t test, 1-way ANOVA or chi-square analysis, as appropriate. Given the case-control study design, the association between variables and AF was evaluated using binary logistic regression models. Because there are no established reference values of miRNAs, the expression of the miRNAs of interest was stratified into quartiles or as above/below the median of all values obtained in our cohort. Those variables showing an association with a P <.1 in the univariable model were included in the multivariable analysis. The discriminatory ability of the model was assessed by the area under the receiver operating characteristic curve. Predicted probabilities were calculated at 6 months by generalized linear models using the variables of interest from the multivariable model and plotted according to them. Significance was set at P <.05. Statistical analyses were performed with SPSS Inc (version 26.0 for Windows, Chicago, IL, United States).
RESULTSStudy populationDuring the study period, a total of 530 patients aged 50 to 89 years were admitted with ischemic stroke. After a complete work-up, 74 patients with a CrS were eligible for the study, of which 10 were subsequently excluded. Therefore, the study population comprised 64 patients with a definite diagnosis of CrS under continuous monitoring with an insertable cardiac monitor implanted before discharge.
The clinical characteristics of the final study population are summarized in table 1. Mean age was 75±11 years, and 29 patients (45%) were women. The prevalence of cardiovascular risk factors was high. Eleven patients (17%) had had a prior stroke or transient ischemic attack. Cardiac evaluation during admission showed normal left ventricle and left atrium dimensions and function in the global population. Stroke characteristics, treatment and severity are presented in table 1.
Characteristics of the study population
Overall(n=64) | CrS-SR(n=36) | CrS-AF(n=28) | P | |
---|---|---|---|---|
Baseline characteristics and past medical history | ||||
Age, y | 74.6 (10.8) | 73.0 (11.8) | 76.7 (9.2) | .180 |
Female sex | 29 (45.3) | 18 (50.0) | 11 (39.3) | .454 |
BMI, kg/m2 | 27.5 (4.5) | 27.4 (3.8) | 27.6 (5.4) | .904 |
Hypertension | 45 (70.3) | 28 (77.8) | 17 (60.7) | .173 |
Diabetes mellitus | 13 (20.3) | 9 (25.0) | 4 (14.3) | .359 |
Hyperlipidemia | 35 (54.7) | 21 (58.3) | 14 (50.0) | .615 |
Current smoker | 8 (12.5) | 5 (13.9) | 3 (10.7) | 1.000 |
Peripheral artery disease | 11 (17.2) | 4 (11.1) | 7 (25.0) | .188 |
Coronary artery disease | 3 (4.7) | 1 (2.8) | 2 (7.1) | .577 |
Prior stroke/TIA | 11 (17.2) | 5 (13.9) | 6 (21.4) | .513 |
CHA2DS2-VASc | 3.2 (1.8) | 3.2 (1.7) | 3.2 (1.9) | .979 |
Cardiac parameters | ||||
LVDd, mm | 45.3 (4.0) | 44.4 (3.2) | 46.5 (4.6) | .054 |
LVDs, mm | 26.8 (3.8) | 26.0 (3.2) | 27.9 (4.3) | .061 |
LVEF, % | 63.5 (3.2) | 63.9 (3.3) | 63.0 (3.1) | 311 |
LAD, mm | 36.5 (4.5) | 35.3 (4.5) | 37.9 (4.2) | .029 |
LA biplanar area, cm2 | 18.1 (3.2) | 17.1 (2.6) | 19.3 (3.6) | .010 |
LAEF, % | 54.2 (11.2) | 58.5 (10.0) | 48.9 (10.3) | .001 |
Stroke characteristics | ||||
TIA | 11 (17.2) | 6 (16.7) | 5 (17.9) | 1.000 |
Oxford scale, available for 60 patients | ||||
TACI | 19 | 12 | 7 | .886 |
PACI | 28 | 14 | 14 | |
LACI | 2 | 1 | 1 | |
POCI | 11 | 7 | 4 | |
NIHSS (admission) | 5 [1.75-12.25] | 3 [1-13] | 5.5 [2-11.25] | .193 |
Fibrinolysis | 20 (31.3) | 11 (30.6) | 9 (32.1) | 1.000 |
NIHSS (discharge) | 1 [0-2] | 1 [0-2] | 1 [0-2.25] | .864 |
AF, atrial fibrillation; BMI, body mass index; CrS, cryptogenic stroke; LAD, left atrial diameter; LAEF, left atrial ejection fraction; LVDd/LVDs, end-diastolic/end-systolic left ventricular diameter; LVEF, left ventricular ejection fraction; NIHSS, National institute of Health Stroke Scale; TACI/PACI/POCI/LACI, total anterior/partial anterior/posterior/lacunar circulation infarct.; TIA, transient ischemic attack.
The results are expressed as absolute numbers, No. (%), or median [interquartile range].
Of the 64 patients, 28 had AF (25 paroxysmal, 3 persistent) within the first 6 months, constituting the CrS-AF group. Oral anticoagulation was initiated in all patients. Patients with AF had a median of 4.3 (0.6-2) AF episodes per month, with a median total AF time of 183.9minutes (23.7-775.6), representing 0.071% (0.009-0.30) of the monitoring time. The remaining 36 patients showed persistent SR (CrS-SR group). The characteristics of patients with AF and those in SR are summarized in table 1. Patients in the CrS-AF group tended to have higher ventricular dimensions and had higher atrial dimensions, together with lower left atrial ejection fraction compared with patients in the CrS-SR group.
miRNA studyThe characteristics of the patients included in the discovery phase (9 patients in CrS-AF, 9 in CrS-SR, and 9 in CES-AF) are summarized in . All groups were sex- and age-matched, with 4 women and a mean age of 75 years. Sixteen miRNAs were differentially expressed in CrS-AF vs CrS-SR (see complete list in ), of which 8 emerged as potential candidates based on their plasma stability. These are represented in figure 2A (shadowed area). All 8 candidates showed relatively consistent expression between CrS-AF and CES-AF compared with controls, and 5 of them (miR-744-5p, miR-1-3p, miR-377-3p, miR-425-5p, and miR-19b-3p) were also significantly different between CES-AF and CrS-SR patients, suggesting that these could be potential indicators of the presence of an AF-favoring environment, irrespective of whether the arrhythmia had been documented or remained subclinical. Expression values of the 8 candidate miRNAs in the Open Array assay are depicted in figure 2B.
A: results of the Open Array study. Out of the 854 miRNAs screened in all 3 study groups (CrS-SR, CrS-AF, and CES-AF), 8 were differentially expressed between CrS-AF and CrS-SR (gray-shadowed area), of which 5 were also differentially expressed between CES-AF and CrS-SR (in bold). Overexpressed miRNAs are presented in blue, whereas downregulated miRNAs appear in orange. B: results of the 8 miRNAs that were selected for replication. Data are expressed in Rq (FC) vs the CrS-SR group (control), which was normalized to 1. Specific P values are provided in . C: results of plasma miRNA expression in the replication cohort. miRNA-1-3p was the only candidate that was confirmed to be significantly increased in CrS-AF vs CrS-SR patients. AF, atrial fibrillation; CES, cardioembolic stroke; CrS, cryptogenic stroke; FC, fold change; SR, sinus rhythm.
All 8 candidate miRNAs were replicated in an independent cohort defined by the presence or absence of AF at 6 months (set 2, ). The results are shown in figure 2C. Only the plasma levels of miR-1-3p remained significantly increased in CrS-AF vs CrS-SR patients (2.81-fold increase, P=.014).
Association between miR-1-3p and atrial fibrillation at 6 monthsUsing the global cohort (n=64), we further explored the potential association between miR-1-3p and AF. Since the “normal range” of plasma levels of miR-1-3p has not been, and fold change values do not provide real plasma levels but a value that is proportional to their concentration, the miR-1-3p results were stratified into quartiles (Q1-Q4). As shown in figure 3A, miR-1-3p levels showed a positive association with the risk of AF, where patients in Q4 and Q3 were more likely to show AF at 6 months of follow-up (69% and 56%, respectively) compared with those in the lower quartiles (31% in Q2 and 19% in Q1, P=.017).
A: occurrence of AF according to quartiles of miR-1-3p in the global population. B: association between miR-1-3p values and AF burden. Percentage of time in AF (top right) and number of AF episodes per month (top left) are presented according to miR-1-3p quartiles. On the bottom panel, distribution of miR-1-3p quartiles among patients with no AF, low AF burden and high AF burden is depicted. AF, atrial fibrillation.
Moreover, miR-1-3p levels appeared moderately associated with AF burden in our cohort. figure 3B depicts the percentage of time in AF (top, right) and the number of AF episodes per month (top, left) that were recorded among patients in the 4 miR-1-3p quartiles throughout the 6 months of follow-up. AF burden measured by both parameters seemed greater among patients in higher miR-1-3p quartiles. The bottom panel of figure 3B represents the distribution of miR-1-3p quartiles among patients with no AF, low AF burden, and high AF burden. Notably, more than half of patients with high AF burden had miR-1-3p values in Q4, whereas none had miR-1-3p values in Q1.
Indicators of subclinical atrial fibrillation at 6 monthsWe then evaluated whether miR-1-3p, in combination with clinical data, could provide early guidance in the identification of CrS patients with subclinical AF. For better applicability, we grouped all miR-1-3p values into above (Q3 + Q4) or below (Q1 + Q2) the median.
Table 2 summarizes the results of logistic regression analyses. Only 2 parameters remained independently associated with AF in the multivariable analysis: a) left atrial ejection fraction, associated with an 11% relative reduction in the AF risk per unit of increase; and b) high plasma levels of miR-1-3p, where values above the median were associated with an 8.5-fold increase in the risk of AF at 6 months. The model including both variables showed a discriminatory ability, evaluated by the area under the receiver operating characteristic curve, of 0.860 (0.760–0.960, P <.001). Figure 4 depicts the estimated predicted probabilities of AF documentation at 6 months after stroke according to these variables.
Clinical and biological variables associated with atrial fibrillation at 6 months
Univariable analysis | Multivariable analysis | |||||
---|---|---|---|---|---|---|
OR | 95%CI | P | OR | 95%CI | P | |
Age, years | 1.04 | 0.98-1.09 | .183 | -- | -- | -- |
Sex, men | 0.65 | 0.24-1.76 | .394 | -- | -- | -- |
LVDd, mm | 1.15 | 1.00-1.32 | .050 | 1.12 | 0.96-1.30 | .169 |
LVDs, mm | 1.14 | 0.99-1.32 | .066 | 0.96 | 0.72-1.28 | .788 |
Left atrium biplanar area, cm2 | 1.25 | 1.05-1.50 | .014 | 0.92 | 0.68-1.24 | .568 |
LAEF, % | 0.91 | 0.85-0.97 | .003 | 0.89 | 0.82-0.96 | .003 |
miR-1-3p> median | 4.55 | 1.54-13.42 | .006 | 8.51 | 2.05-35.25 | .003 |
95%CI, 95% confidence interval; LAEF, left atrial ejection fraction; LVDd/LVDs, end-diastolic/end-systolic left ventricular diameter; OR, odds ratio.
Predicted probabilities of AF risk at 6 months in the global cohort according to LAEF and miR-1-3p levels [expressed as above (red) or below (blue) the median of all values]. Shadowed areas represent 95% confidence intervals. AF, atrial fibrillation; LAEF, left atrial ejection fraction.
To further evaluate the potential clinical impact of plasma miR-1-3p in the assessment of subclinical AF in patients with CrS, we investigated the association between miR-1-3p and the presence of AF at 12 months. Four additional patients developed AF between 6 and 12 months of follow-up (figure 1). miR-1-3p levels were significantly higher in patients showing AF at 12 months than in those with persistent SR (figure 5A). Moreover, miR-1-3p quartiles were positively associated with the presence of AF (figure 5B). Finally, by logistic regression analysis, both miR-1-3p above the median and left atrial ejection fraction remained independently associated with the presence of AF at 12 months (table 3).
Association between miR-1-3p levels and AF at 12 months. A: results of plasma miRNA expression in the replication cohort, with 23 patients in SR and 23 having had at least 1 episode of AF. B: occurrence of AF at 12 months according to quartiles of miR-1-3p in the global population. AF, atrial fibrillation; CrS: cryptogenic stroke; FC, fold change; SR, sinus rhythm.
Clinical and biological variables associated with atrial fibrillation at 12 months
Univariable analysis | Multivariable analysis | |||||
---|---|---|---|---|---|---|
OR | 95%CI | P | OR | 95%CI | P | |
Age, y | 1.06 | 1.01-1.12 | .032 | 1.04 | 0.96-1.12 | .367 |
Male sex | 0.88 | 0.33-2.36 | .802 | -- | -- | -- |
LVDs, mm | 1.13 | 0.98-1.30 | .092 | 1.09 | 0.91-1.32 | .354 |
Left atrium biplanar area, cm2 | 1.32 | 1.09-1.60 | .005 | 1.03 | 0.76-1.40 | .866 |
LAEF, % | 0.87 | 0.80-0.94 | .001 | 0.83 | 0.74-0.93 | .001 |
miR-1-3p> median | 4.41 | 1.52-12.79 | .006 | 11.91 | 2.40-59.18 | .002 |
95%CI, 95% confidence interval; LAEF, left atrial ejection fraction; LVDs, end-systolic left ventricular diameter; OR, odds ratio.
This study, performed in a selected cohort, suggests that high miR-1-3p levels at the time of admission are associated with subclinical AF in patients with CrS. High miR-1-3p, as well as impaired atrial performance assessed by a low left atrial ejection fraction in an initial echocardiogram, could be indicative of a favoring atrial substrate predisposing to AF and could therefore represent potential markers of occult AF in patients with CrS.
Searching for subclinical AF in patients with CrS remains a matter of major interest given the deep prognostic and therapeutic implications that the arrhythmia carries in this setting.1,3 The reported rates of AF detection in CrS have been increasing in recent years in parallel with the use of more intensive monitoring strategies.3,4 The use of an ultra-early monitoring strategy by means of a predischarge insertable cardiac monitor-implant led to an AF rate of 58% at 31 months in a cohort of patients with thoroughly evaluated CrS,6 highlighting that subclinical AF may underlie more CrS cases than previously thought.1,3,4 However, even for the best possible scenario, identifying AF by monitoring strategies comes with the inherent limitation that AF is most often paroxysmal in nature, and therefore its documentation might occur late after the stroke, delaying oral anticoagulation treatment in patients potentially at risk.
The identification of biological and/or clinical markers of subclinical AF that could be present at admission has been investigated in a number of works in recent years. Clinical factors have shown limited predictive value.20 Recently, more promising results have been imported in an extensive study of the left atrium by imaging studies.21,22 In parallel, several biomarkers of subclinical AF have been investigated. N-terminal pro-B-type natriuretic peptide (NT-pro-BNP) and BNP have provided the most consistent results,23,24 with the former potentially outperforming the latter.25 Molecules related to hypercoagulability such as D-dimer and antithrombin-III have shown lower sensitivities and specificities.23 A recent pilot study in CrS patients assessing 184 potential biomarkers simultaneously found the greatest association of AF with proteins related to inflammation, opening up new lines for future research.26
A limitation of these studies, however, comes from the strategy used for AF documentation, which was either intermittent (ECG and Holter-based)22–24 or short-term (<1-month monitoring)25,26. In the present work, we sought to ensure that the arrhythmia was certainly present or otherwise absent in all patients in our cohort, and thus all of them underwent continuous long-term monitoring by means of a predischarge insertable cardiac monitor implant. We defined AF by a compatible recording of> 1minute duration. Different thresholds have been used for AF definition in the setting of CrS, ranging from 30seconds5 to 2minutes.27,28 We chose a threshold of 1minute based on a previous publication showing that initiation of oral anticoagulation according to this definition was associated with a reduction in the risk of new stroke.6 Our primary endpoint was the occurrence of AF within the first 6 months after the stroke. This timeframe has been previously used in pivotal studies on CrS such as the CRYSTAL study.5 For a biomarker study, a 6-month period seems adequate to better establish an association between the biomarker and the arrhythmia, avoiding incidental confounding factors. However, in an attempt to provide greater clinical relevance, we performed a secondary analysis at 12 months, which also confirmed the association between miR-1-3p and AF.
Another strength of our study is that, unlike previous studies, we performed a high-throughput investigation. We chose to assess the profile of circulating miRNAs given that, at the tissue level, these molecules have been extensively involved in the promotion of AF-favoring atrial remodeling,8 and, at the plasma level, some have shown good tissue-correlation.9,10 Importantly, because some inconsistency has been described regarding circulating miRNA profiles in the literature,8 we further included an additional set of patients presenting with concomitant stroke and AF to assist in the selection of candidate miRNAs most likely to be associated with AF, either clinical or subclinical.
We found that patients with CrS and subsequent AF had higher plasma levels of miR-1-3p. miR-1-3p is a member of the muscle specific miR-1 family, which has been consistently implicated in cardiac disease and particularly in arrhythmogenesis.29 The proarrhythmic effects of miR-1 are well endorsed by previous publications showing that miR-1 may promote triggering mechanisms (by increasing inward Ca2+ current and enhancing the frequency of spontaneous Ca2+ sparks),30 but also generate a favorable substrate facilitating anatomical and functional re-entry (by depolarizing cytoplasmic membrane and slowing conduction through repression of KCNJ2 and GJA1, respectively).31 All these mechanisms are known to participate in AF promotion and maintenance.7 Furthermore, a previous work reported that 1-week atrial tachypacing in rabbits increased miR-1 expression and AF inducibility.32 Previous studies, however, refer to miR-1 overexpression at the cardiac level. Little information exists on the role of plasma miR-1-3p. A recent pilot study found higher miR-1-3p plasma levels in patients with AF and cardioembolic stroke compared with those in AF without stroke.33 Our results suggest that high miR-1-3p levels could also be indicative of subclinical AF in CrS patients. Moreover, miR-1-3p levels showed a potential association with AF burden in our population. While waiting to be confirmed by future studies with larger populations of patients, these results further support a role of miR-1-3p as a marker of atrial arrhythmogenicity and AF risk in patients with CrS.
LimitationsOur work has several limitations. The sample size was small, particularly in the replication cohort, but included an invaluable population of consecutive patients thoroughly evaluated under continuous monitoring. Even so, the final population was not significantly smaller than that used in previous studies on circulating miRNAs using high-throughput methodology.18 Our population included men and women aged between 50 and 89 years. Therefore, the present results should not be extrapolated to other age groups. This study was performed in a single tertiary hospital and lacks external validation in other cohorts, which would be most desirable to definitely establish the role of miR-1-3p as a biomarker of subclinical AF in patients with CrS. Finally, we cannot confirm that high circulating levels of miR-1-3p reflect high miR-1-3p expression at the atrial level, or the presence of an AF-favoring substrate. However, our results point to miR-1-3p as a potential biomarker of subclinical AF regardless its tissue expression or the underlying atrial substrate. Whether high plasma miR-1-3p levels may be directly responsible for AF promotion in the context of CrS, reach true clinical applicability, and even might provide guidance for early oral anticoagulation initiation should be evaluated after external validation in future studies.
CONCLUSIONSOur results suggest that plasma levels of miR-1-3p, a gene regulator consistently involved in cardiac arrhythmogenesis, could be elevated in CrS patients with subsequent AF, especially among those with high arrhythmic burden. Our results preliminary indicate that miR-1-3p could be a novel biomarker that, together with clinical parameters such as an impaired left atrial ejection fraction, could help identify patients with CrS and high risk of occult AF.
- -
Among patients with CrS, there is need to promptly identify patients with subclinical AF.
- -
Long-term continuous monitoring strategies have shown better diagnostic yield than conventional strategies, but are still suboptimal.
- -
Several selected biomarkers have also been proposed, with limited predictive ability.
- -
Unlike previous series, this study included a cohort that was thoroughly evaluated with a predischarge insertable cardiac monitor and high-throughput determination of microRNAs.
- -
Plasma levels of miR-1-3p, a gene regulator linked to arrhythmogenesis, were higher in patients with CrS and subsequent AF than in those in SR. Our study suggests for the first time the potential usefulness of a regulator involved in AF pathophysiology as a plasma biomarker.
- -
Our preliminary data suggest that, together with a low left atrial ejection fraction, high miR-1-3p levels could help identify, as early as at the time of admission and with good predictive ability, patients with CrS and high risk of occult AF.
Supported by Instituto de Salud Carlos III–FIS (Fondo de Investigación Sanitaria, PI13/01830) and Societat Catalana de Cardiologia 2016 (both awarded to BB); Spain's Ministry of Health (FEDER, RD16/0019/0002- INVICTUS-PLUS) awarded to the Neurology Department, Hospital del Mar. E. Giralt-Steinhauer received funding from the Instituto de Salud Carlos III (JR18/00004).
AUTHORS’ CONTRIBUTIONSAll authors listed in the article made a substantial contribution to the concept and design, acquisition of data, or analysis and interpretation of the results of the present study. Additionally, all had the opportunity to draft and/or critically revise the content of this manuscript and approved the current version to be published. B. Benito and A. García-Elías contributed equally to this work.
CONFLICTS OF INTERESTBiotronik, Inc kindly provided 35 of the implantable cardiac monitors (Biomonitor) required for this study. The authors have no relevant financial or nonfinancial conflicts of interest to disclose.
Supplementary data associated with this article can be found in the online version available at https://doi.org/10.1016/j.rec.2021.12.001